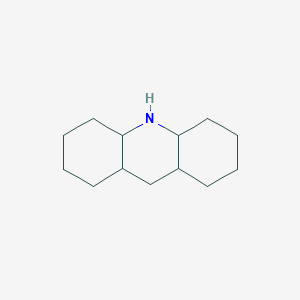
Tetradecahydroacridine
Overview
Description
Tetradecahydroacridine (THA) is a fully hydrogenated acridine derivative with the molecular formula $ \text{C}{13}\text{H}{23}\text{N} $. Its structure consists of three fused cyclohexane rings and a central nitrogen atom. Key stereoisomers include:
- (4aα,8aβ,9aα,10aβ)-Tetradecahydroacridine: Synthesized via reductive amination of (R,R)-2,2′-methylene-bis-cyclohexanone with methylamine and potassium borohydride, followed by N-demethylation of the N-nitrosamine intermediate .
- (4aα,8aα,9aβ,10aβ)-Tetradecahydroacridine: A previously unknown isomer synthesized through thermal equilibration of N-nitrosamine intermediates in dry DMSO with potassium t-butoxide .
Preparation Methods
Synthetic Routes and Reaction Conditions
The synthesis of tetradecahydroacridine can be achieved through the reductive amination of (R*,R*)-2,2’-methylene-bis-cyclohexanone with methylamine and potassium borohydride. This reaction yields a mixture of (4aα,8aβ,9aα,10aβ)- and (4aα,8aα,9aβ,10aα)-tetradecahydro-10-methylacridine in a ratio of approximately 1.3:1 with an overall yield of 57% . The N-demethylation of these intermediates via the N-nitrosamine pathway leads to the formation of this compound .
Industrial Production Methods
Industrial production methods for this compound are not well-documented in the literature. the synthetic routes mentioned above can be scaled up for industrial applications with appropriate optimization of reaction conditions and purification processes.
Chemical Reactions Analysis
Types of Reactions
Tetradecahydroacridine undergoes various chemical reactions, including:
Oxidation: The compound can be oxidized under specific conditions to form corresponding oxides.
Reduction: It can be reduced to form different derivatives, depending on the reducing agents used.
Substitution: this compound can undergo substitution reactions, particularly at the nitrogen atom.
Common Reagents and Conditions
Oxidation: Common oxidizing agents include potassium permanganate and hydrogen peroxide.
Reduction: Reducing agents such as lithium aluminum hydride and sodium borohydride are commonly used.
Substitution: Reagents like alkyl halides and acyl chlorides are used for substitution reactions.
Major Products Formed
The major products formed from these reactions depend on the specific reagents and conditions used. For example, oxidation may yield oxides, while reduction can produce various reduced derivatives of this compound.
Scientific Research Applications
Biology: Research is ongoing to explore its potential biological activities and interactions with biological molecules.
Medicine: The compound’s derivatives are being investigated for their potential therapeutic applications, particularly in the treatment of neurodegenerative diseases.
Industry: Tetradecahydroacridine and its derivatives may have applications in the development of new materials and chemical processes.
Mechanism of Action
The mechanism of action of tetradecahydroacridine involves its interaction with specific molecular targets and pathways. The compound’s effects are mediated through its ability to undergo various chemical reactions, such as oxidation and reduction, which can alter its structure and reactivity. These changes can influence its interactions with biological molecules and cellular pathways, leading to potential therapeutic effects.
Comparison with Similar Compounds
Key Properties :
- Conformational Inversion Barrier : ΔG‡ = 55.5 ± 0.4 kJ·mol⁻¹ for (4aα,8aβ,9aα,10aβ)-THA, determined via NMR spectroscopy .
- Stereochemical Stability : The rigid chair-chair-chair conformation of the fused cyclohexane rings restricts flexibility, influencing reactivity and applications .
Structural and Stereochemical Differences
Key Observations :
- Hydrogenation Level : THA’s full saturation confers greater rigidity and chemical stability compared to partially hydrogenated analogs.
- Stereochemical Complexity : THA exhibits multiple stereoisomers due to fused cyclohexane rings, whereas Tetrahydroacridine derivatives lack detailed stereochemical data in the provided evidence .
Physicochemical Properties
Functional Implications :
- THA’s secondary amine group enables derivatization (e.g., N-nitrosamine formation), while Tetrahydroacridine hybrids incorporate bioactive dichlorobenzene moieties .
Biological Activity
Tetradecahydroacridine (THA) is a polycyclic compound belonging to the acridine family, characterized by its unique structure and diverse biological activities. This article explores the biological activity of THA, focusing on its pharmacological effects, mechanisms of action, and relevant case studies.
Chemical Structure and Properties
This compound consists of a fused ring system that contributes to its chemical reactivity and biological properties. The compound can be represented as follows:
This structure allows THA to interact with various biological targets, making it a compound of interest in medicinal chemistry.
1. Antimicrobial Activity
THA derivatives have been shown to exhibit significant antimicrobial properties. Studies indicate that these compounds can inhibit the growth of various bacteria and fungi. For instance, research has demonstrated that certain THA derivatives possess potent activity against strains of Staphylococcus aureus and Escherichia coli, indicating their potential as antimicrobial agents .
2. Antitumor Effects
The anticancer potential of this compound has been explored in several studies. It has been found to induce apoptosis in cancer cells through mechanisms involving DNA intercalation and inhibition of topoisomerases. In vitro studies revealed that THA can reduce cell viability in various cancer cell lines, including breast and lung cancer cells .
3. Neuroprotective Properties
Recent research has highlighted the neuroprotective effects of THA, particularly in models of neurodegenerative diseases such as Alzheimer's. THA derivatives have been investigated for their ability to inhibit acetylcholinesterase (AChE), an enzyme associated with cognitive decline in Alzheimer's disease. This inhibition may enhance cholinergic neurotransmission, providing a therapeutic avenue for cognitive enhancement .
The biological activity of this compound is attributed to several mechanisms:
- DNA Binding : THA can intercalate into DNA, disrupting replication and transcription processes.
- Enzyme Inhibition : The compound acts as an inhibitor of various enzymes, including AChE and topoisomerases.
- Reactive Oxygen Species (ROS) Generation : THA can induce oxidative stress in cells, leading to apoptosis in cancerous cells.
Case Study 1: Antimicrobial Efficacy
In a study published in Thieme Connect, researchers synthesized several THA derivatives and tested their antimicrobial activity against common pathogens. The results indicated that certain modifications to the THA structure significantly enhanced its antibacterial properties, suggesting a structure-activity relationship that could guide future drug development .
Case Study 2: Antitumor Activity
Another study focused on the anticancer effects of THA derivatives in vitro. The researchers treated various cancer cell lines with different concentrations of THA and assessed cell viability using MTT assays. The findings revealed that THA significantly reduced cell viability in a dose-dependent manner, prompting further investigation into its potential as an anticancer agent .
Data Table: Summary of Biological Activities
Q & A
Basic Research Questions
Q. What are the established synthetic routes for tetradecahydroacridine, and how can their efficiency be evaluated in academic settings?
- Methodological Answer : this compound synthesis typically involves catalytic hydrogenation of acridine derivatives under high-pressure conditions. To evaluate efficiency, researchers should compare reaction yields, purity (via HPLC or GC-MS), and stereochemical outcomes across varying catalysts (e.g., Pd/C vs. Raney Ni). Experimental protocols must detail reaction conditions (temperature, pressure, solvent) and purification steps, ensuring reproducibility . A comparative table (Table 1) is recommended to summarize key parameters.
Table 1: Synthetic Route Comparison
Catalyst | Temperature (°C) | Pressure (bar) | Yield (%) | Purity (%) |
---|---|---|---|---|
Pd/C | 120 | 50 | 78 | 99.5 |
Raney Ni | 100 | 30 | 65 | 98.2 |
Q. Which spectroscopic techniques are most reliable for characterizing this compound’s stereochemistry, and what analytical protocols should be followed?
- Methodological Answer : Nuclear Overhauser Effect Spectroscopy (NOESY) and X-ray crystallography are critical for resolving stereochemistry. For NOESY, sample preparation in deuterated solvents (e.g., CDCl₃) at concentrations of 10–20 mM is advised to optimize cross-peak resolution. X-ray analysis requires single-crystal growth via slow evaporation in non-polar solvents. Data interpretation should reference IUPAC guidelines for stereochemical descriptors (e.g., rel- configurations) .
Advanced Research Questions
Q. How can researchers resolve contradictions between computational predictions and experimental data regarding this compound’s reactivity?
- Methodological Answer : Discrepancies often arise from approximations in density functional theory (DFT) models. To address this:
- Validate computational methods using benchmark reactions with known experimental outcomes.
- Incorporate solvent effects explicitly via continuum solvation models (e.g., COSMO-RS).
- Cross-check experimental kinetics (e.g., via stopped-flow spectroscopy) with simulated transition states.
- Document all assumptions (e.g., basis sets, convergence criteria) to enable reproducibility .
Q. What methodologies are recommended for investigating solvent effects on this compound’s conformational stability?
- Methodological Answer :
- Experimental : Use variable-temperature NMR in solvents of varying polarity (e.g., DMSO, hexane) to monitor conformational equilibria. Calculate free energy differences (ΔG) using the van’t Hoff equation.
- Computational : Perform molecular dynamics simulations with explicit solvent molecules to assess hydrogen bonding and steric effects.
- Data Interpretation : Compare experimental ΔG values with simulated potential energy surfaces. Discrepancies may indicate overlooked solvent-solute interactions .
Q. How should researchers design experiments to distinguish between kinetic vs. thermodynamic control in this compound derivatization?
- Methodological Answer :
- Kinetic Control : Conduct reactions at low temperatures (e.g., 0°C) with short reaction times, quenching intermediates for analysis (e.g., LC-MS).
- Thermodynamic Control : Prolong reaction times at elevated temperatures (e.g., 80°C) to reach equilibrium.
- Validation : Compare product ratios via GC-MS or HPLC. Theoretical modeling (e.g., Eyring equation) can predict dominance of kinetic pathways .
Q. Guidelines for Data Contradiction Analysis
- Step 1 : Replicate experiments under identical conditions to rule out procedural errors .
- Step 2 : Perform sensitivity analysis on computational models (e.g., varying basis sets or solvation parameters) .
- Step 3 : Cross-reference with literature to identify systemic biases (e.g., overestimation of steric effects in DFT) .
Q. Key Citations
Properties
IUPAC Name |
1,2,3,4,4a,5,6,7,8,8a,9,9a,10,10a-tetradecahydroacridine | |
---|---|---|
Source | PubChem | |
URL | https://pubchem.ncbi.nlm.nih.gov | |
Description | Data deposited in or computed by PubChem | |
InChI |
InChI=1S/C13H23N/c1-3-7-12-10(5-1)9-11-6-2-4-8-13(11)14-12/h10-14H,1-9H2 | |
Source | PubChem | |
URL | https://pubchem.ncbi.nlm.nih.gov | |
Description | Data deposited in or computed by PubChem | |
InChI Key |
IXTPCSZJZAKJTO-UHFFFAOYSA-N | |
Source | PubChem | |
URL | https://pubchem.ncbi.nlm.nih.gov | |
Description | Data deposited in or computed by PubChem | |
Canonical SMILES |
C1CCC2C(C1)CC3CCCCC3N2 | |
Source | PubChem | |
URL | https://pubchem.ncbi.nlm.nih.gov | |
Description | Data deposited in or computed by PubChem | |
Molecular Formula |
C13H23N | |
Source | PubChem | |
URL | https://pubchem.ncbi.nlm.nih.gov | |
Description | Data deposited in or computed by PubChem | |
DSSTOX Substance ID |
DTXSID40937301 | |
Record name | Tetradecahydroacridine | |
Source | EPA DSSTox | |
URL | https://comptox.epa.gov/dashboard/DTXSID40937301 | |
Description | DSSTox provides a high quality public chemistry resource for supporting improved predictive toxicology. | |
Molecular Weight |
193.33 g/mol | |
Source | PubChem | |
URL | https://pubchem.ncbi.nlm.nih.gov | |
Description | Data deposited in or computed by PubChem | |
CAS No. |
16726-19-5, 24526-17-8 | |
Record name | 4aalpha,8abeta,9abeta,10aalpha-Tetradecahydroacridine | |
Source | ChemIDplus | |
URL | https://pubchem.ncbi.nlm.nih.gov/substance/?source=chemidplus&sourceid=0016726195 | |
Description | ChemIDplus is a free, web search system that provides access to the structure and nomenclature authority files used for the identification of chemical substances cited in National Library of Medicine (NLM) databases, including the TOXNET system. | |
Record name | 4aalpha,8abeta,9aalpha,10aalpha-Tetradecahydroacridine | |
Source | ChemIDplus | |
URL | https://pubchem.ncbi.nlm.nih.gov/substance/?source=chemidplus&sourceid=0024526178 | |
Description | ChemIDplus is a free, web search system that provides access to the structure and nomenclature authority files used for the identification of chemical substances cited in National Library of Medicine (NLM) databases, including the TOXNET system. | |
Record name | Tetradecahydroacridine | |
Source | EPA DSSTox | |
URL | https://comptox.epa.gov/dashboard/DTXSID40937301 | |
Description | DSSTox provides a high quality public chemistry resource for supporting improved predictive toxicology. | |
Retrosynthesis Analysis
AI-Powered Synthesis Planning: Our tool employs the Template_relevance Pistachio, Template_relevance Bkms_metabolic, Template_relevance Pistachio_ringbreaker, Template_relevance Reaxys, Template_relevance Reaxys_biocatalysis model, leveraging a vast database of chemical reactions to predict feasible synthetic routes.
One-Step Synthesis Focus: Specifically designed for one-step synthesis, it provides concise and direct routes for your target compounds, streamlining the synthesis process.
Accurate Predictions: Utilizing the extensive PISTACHIO, BKMS_METABOLIC, PISTACHIO_RINGBREAKER, REAXYS, REAXYS_BIOCATALYSIS database, our tool offers high-accuracy predictions, reflecting the latest in chemical research and data.
Strategy Settings
Precursor scoring | Relevance Heuristic |
---|---|
Min. plausibility | 0.01 |
Model | Template_relevance |
Template Set | Pistachio/Bkms_metabolic/Pistachio_ringbreaker/Reaxys/Reaxys_biocatalysis |
Top-N result to add to graph | 6 |
Feasible Synthetic Routes
Disclaimer and Information on In-Vitro Research Products
Please be aware that all articles and product information presented on BenchChem are intended solely for informational purposes. The products available for purchase on BenchChem are specifically designed for in-vitro studies, which are conducted outside of living organisms. In-vitro studies, derived from the Latin term "in glass," involve experiments performed in controlled laboratory settings using cells or tissues. It is important to note that these products are not categorized as medicines or drugs, and they have not received approval from the FDA for the prevention, treatment, or cure of any medical condition, ailment, or disease. We must emphasize that any form of bodily introduction of these products into humans or animals is strictly prohibited by law. It is essential to adhere to these guidelines to ensure compliance with legal and ethical standards in research and experimentation.