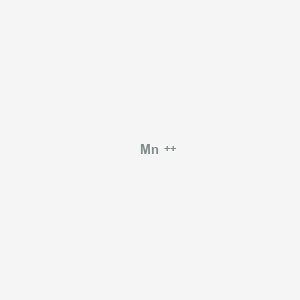
Manganese(2+)
- Click on QUICK INQUIRY to receive a quote from our team of experts.
- With the quality product at a COMPETITIVE price, you can focus more on your research.
Overview
Description
Manganese(2+), or Mn²⁺, is a divalent cation of manganese, a transition metal with atomic number 23. It is essential in biological systems, serving as a cofactor for enzymes such as manganese superoxide dismutase (SOD2), which mitigates oxidative stress by converting superoxide radicals into hydrogen peroxide . Industrially, Mn²⁺ is utilized in catalysts, battery materials (e.g., lithium-rich manganese oxides), and hybrid organic-inorganic nanomaterials . Its ionic radius (0.83 Å) closely resembles that of Ca²⁺ (1.00 Å), enabling it to mimic calcium in biological processes, such as osteoblast mineralization and neuronal signaling . However, excessive Mn²⁺ accumulation in mitochondria leads to neurotoxicity, resembling Parkinson’s disease, due to disrupted redox homeostasis .
Q & A
Basic Research Questions
Q. What spectroscopic methods are most effective for detecting Mn²⁺ in aqueous solutions, and how should researchers validate their accuracy?
- Methodological Answer : Use UV-Vis spectroscopy (for d-d transitions in the visible range) and Electron Paramagnetic Resonance (EPR) spectroscopy (to detect unpaired electrons in Mn²⁺). Calibrate instruments using standard Mn²⁺ solutions of known concentrations. Validate via cross-referencing with Inductively Coupled Plasma Mass Spectrometry (ICP-MS) for quantitative accuracy . Control for pH and ionic strength, as these factors influence Mn²⁺ speciation and spectral profiles .
Q. How can researchers mitigate interference from Fe²⁺ or Ca²⁺ when quantifying Mn²⁺ in complex matrices?
- Methodological Answer : Employ selective chelators (e.g., EDTA for Ca²⁺ masking) or ion-selective electrodes. Use separation techniques like ion-exchange chromatography or solvent extraction prior to analysis. Validate selectivity via spike-recovery experiments in the presence of interfering ions .
Q. What are best practices for sample preparation in Mn²⁺ stability studies under aerobic vs. anaerobic conditions?
- Methodological Answer : For aerobic studies, use buffered solutions with antioxidants (e.g., ascorbic acid) to minimize oxidation. For anaerobic work, conduct experiments in gloveboxes (O₂ < 1 ppm) and validate conditions using redox-sensitive probes. Document temperature, pH, and ionic strength meticulously to ensure reproducibility .
Advanced Research Questions
Q. How should researchers design experiments to study the redox behavior of Mn²⁺ in biological systems under varying pH conditions?
- Methodological Answer :
- Step 1 : Use cyclic voltammetry with a three-electrode system to measure redox potentials across pH 4–8.
- Step 2 : Couple with spectroscopic monitoring (e.g., X-ray Absorption Spectroscopy, XAS) to track Mn oxidation state changes.
- Step 3 : Model kinetics using Marcus theory, accounting for proton-coupled electron transfer (PCET) mechanisms. Address contradictions in literature by standardizing buffer systems and reporting dissolved O₂ levels .
Q. What statistical approaches resolve contradictions in reported Mn²⁺ binding affinities for ligands like citrate or humic acids?
- Methodological Answer :
- Meta-analysis : Aggregate datasets from multiple studies, normalizing for ionic strength and temperature.
- Error Analysis : Apply Monte Carlo simulations to quantify uncertainty from instrumental noise or fitting algorithms.
- Validation : Compare isothermal titration calorimetry (ITC) and fluorescence quenching data to identify systematic biases. Report confidence intervals and effect sizes to clarify discrepancies .
Q. How can computational models predicting Mn²⁺ interactions with biological molecules be validated experimentally?
- Methodological Answer :
- Docking Simulations : Use DFT or MD simulations to predict binding sites on proteins (e.g., Mn²⁺ in photosystem II).
- Experimental Validation : Employ site-directed mutagenesis to alter predicted binding residues, then measure Mn²⁺ affinity via equilibrium dialysis or microscale thermophoresis. Cross-validate with EXAFS to confirm coordination geometry .
Q. Data Analysis & Reprodubility
Q. How should researchers handle large datasets from Mn²⁺ speciation studies while maintaining reproducibility?
- Methodological Answer :
- Raw Data : Archive in appendices or supplementary materials, adhering to FAIR principles (Findable, Accessible, Interoperable, Reusable).
- Processing : Use open-source tools (e.g., Python’s SciPy) for baseline correction and peak fitting in spectroscopic data. Document code and parameters in repositories like GitHub .
Q. What metrics should be reported to ensure transparency in Mn²⁺ toxicity studies using cell cultures?
- Methodological Answer :
- Dosimetry : Report Mn²⁺ concentrations in media, accounting for precipitation or adsorption.
- Controls : Include positive (e.g., H₂O₂ exposure) and negative (metal-free media) controls.
- Statistical Rigor : Use ANOVA with post-hoc tests; report effect sizes, p-values, and power analysis to avoid Type I/II errors .
Q. Ethical & Methodological Compliance
Q. How can researchers ensure ethical data collection in studies involving Mn²⁺ exposure in animal models?
- Methodological Answer : Follow institutional guidelines for humane endpoints and sample sizes (e.g., ARRIVE 2.0). Use non-invasive imaging (MRI) to minimize sacrifices. Disclose conflicts of interest and funding sources in metadata .
Q. What steps are critical for replicating Mn²⁺-catalyzed reaction mechanisms reported in high-impact journals?
- Methodological Answer :
- Reagent Purity : Use trace-metal-grade chemicals; validate via ICP-MS.
- Detailed Protocols : Share step-by-step workflows, including stirring rates, degassing methods, and catalyst aging effects.
- Peer Review : Publish negative results or failed replications to address publication bias .
Comparison with Similar Compounds
Comparison with Divalent Transition Metal Ions
Key Findings :
- Mn²⁺ exhibits higher redox versatility than Zn²⁺ or Ca²⁺, enabling roles in oxidative stress regulation .
- Mn²⁺ competes with Ca²⁺ in voltage-gated channels, affecting osteoblast activity and neuronal signaling .
Comparison with Other Manganese Oxidation States
Key Findings :
- Mn²⁺ compounds (e.g., MnCl₂) are more soluble and stable in aqueous systems than Mn⁴⁺ oxides (e.g., MnO₂), which dominate battery cathodes .
- Mn²⁺ in silicomanganese slag (MnSiO₃) is less reactive than pure Mn²⁺ salts, impacting metallurgical applications .
Ligand Binding and Complexation
Mn²⁺ forms stable complexes with diverse ligands, including:
- Inorganic ligands: Thiocyanate (Mn(SCN)₂), nitrate (Mn(NO₃)₂) .
- Organic ligands : Citrate, aspartate, and proteins (e.g., casein) via carboxylate and phosphate groups .
Table 3: Stability Constants (log K) of Metal-Ligand Complexes
Ligand | Mn²⁺ | Fe²⁺ | Zn²⁺ |
---|---|---|---|
Citrate | 4.3 | 3.5 | 4.9 |
Oxalate | 5.2 | 4.6 | 5.7 |
Phosphate | 2.1 | 1.8 | 2.5 |
Key Findings :
- Mn²⁺ binds weaker to phosphate than Zn²⁺, influencing its bioavailability in soils and biological systems .
- Thiocyanate complexes (e.g., Mn(SCN)₂) are less stable than analogous Fe²⁺ complexes, limiting their use in catalysis .
Catalytic Performance vs. Rhenium Compounds
In the catalytic decomposition of KClO₃:
- Mn²⁺ catalysts (e.g., MnO) show higher activity than Re compounds (e.g., Re₂O₇) due to favorable redox cycling (Mn²⁺ ↔ Mn³⁺) .
- Mn²⁺-based catalysts achieve 85% KClO₃ conversion at 300°C, outperforming Re catalysts by 20% under identical conditions .
Preparation Methods
Co-Precipitation Method for Manganese(II) Oxide Nanoparticles
The co-precipitation technique is widely employed for synthesizing manganese oxide nanoparticles, particularly due to its cost-effectiveness and simplicity. In a representative procedure, a 0.03 M aqueous solution of manganous sulfate (MnSO₄·H₂O) is reacted with 0.009 M sodium hydroxide (NaOH) at 60°C under constant stirring for two hours . The resultant brown precipitate is centrifuged, dried at 100°C, and calcined at 500°C for two hours to yield crystalline manganese oxide nanoparticles .
Key advantages of this method include:
-
Particle size control : Adjusting NaOH concentration or stirring rate modulates nanoparticle dimensions.
-
Homogeneity : Uniform mixing ensures consistent surface states and phase purity .
-
Scalability : The process is amenable to industrial-scale production without specialized equipment.
Characterization via UV-Vis spectroscopy and FTIR confirms the formation of Mn³O₄ phases, though Mn²⁺-containing intermediates are transiently stabilized during precipitation . Challenges include potential oxidation to higher-valent manganese oxides if calcination exceeds 500°C.
Acid-Metal Reaction for Manganese(II) Salts
Soluble Mn²⁺ salts, such as MnSO₄, are conventionally prepared by reacting manganese metal with dilute acids. For instance, manganese reacts with sulfuric acid (H₂SO₄) as follows:
2\text{SO}4(\text{aq}) \rightarrow \text{MnSO}4(\text{aq}) + \text{H}2(\text{g})Mn(s)+H2SO4(aq)→MnSO4(aq)+H2(g)
Excess manganese ensures complete neutralization of the acid, preventing residual H⁺ from concentrating during crystallization . The solution is filtered to remove unreacted metal and evaporated to isolate MnSO₄ crystals .
Operational considerations :
-
Reactivity : Manganese’s moderate reactivity necessitates dilute acids and elevated temperatures (50–80°C) for practical reaction rates.
-
Impurity management : Byproducts like MnS in sulfuric acid reactions require post-synthesis washing with hot water .
This method yields high-purity Mn²⁺ salts but is less feasible for noble metals, which require concentrated acids and aggressive conditions.
Disproportionation and Ozonation of Manganese(III) Oxides
A patented process for synthesizing manganese dioxide (MnO₂) from Mn(III)-containing oxides indirectly elucidates Mn²⁺ intermediacy . Treating Mn₂O₃ with 20% perchloric acid (HClO₄) at 60°C induces disproportionation:
2\text{O}3 + 2\text{HClO}4 \rightarrow \text{MnO}2 + \text{Mn(ClO}4\text{)}2 + \text{H}_2\text{O}Mn2O3+2HClO4→MnO2+Mn(ClO4)2+H2O
The resultant Mn²⁺ perchlorate is subsequently ozonated at 50–80°C, oxidizing Mn²⁺ to MnO₂ . While the final product is MnO₂, halting the reaction post-disproportionation allows isolation of Mn²⁺ salts.
Critical parameters :
-
Acid concentration : 20–40% HClO₄ optimizes Mn²⁺ yield while minimizing side reactions.
-
Ozone dosage : Excess ozone drives complete oxidation, but controlled dosing preserves Mn²⁺ .
This method’s versatility permits phase control (α- vs. γ-MnO₂) via cation additives (e.g., Na⁺ or K⁺), though its primary utility lies in MnO₂ production .
Complexation of Manganese(II) with Organic Ligands
Manganese(II) complexes with tailored coordination geometries are synthesized by reacting MnCl₂·4H₂O with ligands like 2-acetylpyridine N(4)-ethylthiosemicarbazone . In a typical procedure:
-
Ligand synthesis : 2-acetylpyridine and N(4)-ethylthiosemicarbazide are refluxed in ethanol.
-
Complexation : MnCl₂·4H₂O is added to the ligand solution, yielding a precipitate upon cooling .
Characterization insights :
-
Conductivity : Molar conductance values (~10–15 S·cm²·mol⁻¹) confirm non-electrolytic behavior .
-
FTIR : Ligand-to-metal charge transfer bands at 400–600 cm⁻¹ verify coordination .
Such complexes are pivotal in catalysis and magnetism but require stringent anhydrous conditions to prevent hydrolysis.
Comparative Analysis of Manganese(II) Preparation Methods
Properties
CAS No. |
16397-91-4 |
---|---|
Molecular Formula |
Mn+2 |
Molecular Weight |
54.93804 g/mol |
IUPAC Name |
manganese(2+) |
InChI |
InChI=1S/Mn/q+2 |
InChI Key |
WAEMQWOKJMHJLA-UHFFFAOYSA-N |
SMILES |
[Mn+2] |
Canonical SMILES |
[Mn+2] |
boiling_point |
2061 |
melting_point |
1246 1244°C |
Key on ui other cas no. |
7439-96-5 16397-91-4 |
physical_description |
Solid |
Origin of Product |
United States |
Disclaimer and Information on In-Vitro Research Products
Please be aware that all articles and product information presented on BenchChem are intended solely for informational purposes. The products available for purchase on BenchChem are specifically designed for in-vitro studies, which are conducted outside of living organisms. In-vitro studies, derived from the Latin term "in glass," involve experiments performed in controlled laboratory settings using cells or tissues. It is important to note that these products are not categorized as medicines or drugs, and they have not received approval from the FDA for the prevention, treatment, or cure of any medical condition, ailment, or disease. We must emphasize that any form of bodily introduction of these products into humans or animals is strictly prohibited by law. It is essential to adhere to these guidelines to ensure compliance with legal and ethical standards in research and experimentation.