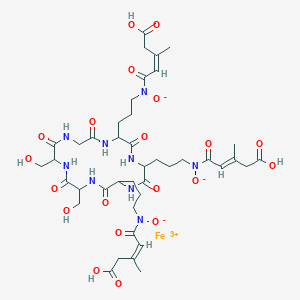
Ferrichrome A
Overview
Description
Ferrichrome A is a siderophore, a type of molecule that binds and transports iron in microorganisms. It belongs to the ferrichrome family, which consists of cyclic hexapeptides that chelate ferric iron through the oxygen atoms of hydroxyl and acyl groups of ornithine residues . This compound was first isolated from the smut fungus Ustilago sphaerogena and is produced by various fungi, including Ustilago maydis .
Preparation Methods
Synthetic Routes and Reaction Conditions: The biosynthesis of Ferrichrome A in Ustilago maydis involves several enzymatic steps. It begins with the synthesis of hydroxymethyl glutaryl-CoA from acetyl-CoA and acetoacetyl-CoA by HMG-CoA synthase (Hcs1). This is followed by the conversion of hydroxymethyl glutaryl-CoA to methyl glutaconyl-CoA via enoyl-CoA hydratase (Fer4). Methyl glutaconyl-CoA is then coupled with hydroxy ornithine, derived from ornithine monoxygenase (Sid1), by hydroxyornithine acylase (Fer5) to yield methylglutaconyl hydroxy ornithine. Finally, cyclization of three methylglutaconyl hydroxy ornithine units with glycine and serine amino acids via nonribosomal peptide synthetase (NRPS) Fer3 produces this compound .
Industrial Production Methods: Industrial production of this compound is not well-documented, as it is primarily studied in a research context. the production process would likely involve fermentation of fungi known to produce this compound, followed by extraction and purification of the compound.
Chemical Reactions Analysis
Types of Reactions: Ferrichrome A primarily undergoes chelation reactions, where it binds ferric iron (Fe³⁺) to form a stable complex. This chelation is crucial for iron transport and bioavailability in microorganisms .
Common Reagents and Conditions: The chelation reaction involves the hydroxyl and acyl groups of the ornithine residues in this compound binding to ferric iron. This process typically occurs under iron-limiting conditions, which induce the production of siderophores like this compound .
Major Products Formed: The major product formed from the chelation reaction is the this compound-iron complex, which facilitates the transport of iron into microbial cells .
Scientific Research Applications
Microbial Iron Uptake
Mechanisms of Iron Acquisition:
Ferrichrome A facilitates iron uptake in microorganisms, particularly in environments where iron is limited. Studies have shown that Pseudomonas aeruginosa utilizes this compound through specific receptors (FiuA and FiuB) for iron transport across its membranes. The energy for this uptake is derived from the inner membrane protein TonB1, highlighting the complex interplay between energy metabolism and siderophore function .
Case Study: Pseudomonas aeruginosa
- Objective: Investigate ferrichrome uptake mechanisms.
- Findings: Approximately 80% of ferrichrome uptake occurs via the FiuA receptor. Mutations in associated proteins significantly impair iron acquisition, demonstrating the importance of ferrichrome in microbial survival under iron-limited conditions .
Plant Growth Promotion
Role in Plant-Microbe Interactions:
this compound has been identified as a growth-promoting factor for certain plants. It enhances nutrient uptake and growth under stress conditions, particularly in high ammonium environments.
Case Study: Fission Yeast Adaptation
- Objective: Assess the effects of ferrichrome on Schizosaccharomyces pombe growth under high ammonium.
- Findings: Ferrichrome mitigated growth suppression caused by high ammonium concentrations, demonstrating its role in nitrogen metabolism regulation . This suggests potential applications in agricultural practices to enhance crop resilience.
Antimicrobial Properties
Siderophore as Antimicrobial Agents:
Recent research has revealed that this compound exhibits antimicrobial properties against various pathogens. Its ability to sequester iron limits the availability of this essential nutrient to competing microorganisms.
Case Study: Lactobacillus casei
- Objective: Explore the tumor-suppressive properties of ferrichrome derived from Lactobacillus casei.
- Findings: Ferrichrome was shown to inhibit tumor growth in vitro, indicating its potential as a therapeutic agent against certain cancers . This discovery opens avenues for further research into its application in cancer treatment.
Biotechnological Applications
Bioproduction and Genetic Engineering:
The biosynthetic pathways for this compound have been characterized, providing insights into genetic engineering possibilities for enhanced production.
Data Table: Siderophore Production Pathways
Organism | Siderophore Produced | Key Genes Involved |
---|---|---|
Omphalotus olearius | This compound | fso1, omo1, ato1 |
Streptomyces sp. | Ferrichrome | abmA, abmB, abmQ |
Pseudomonas aeruginosa | Pyoverdine | fiuA, fiuB |
This table summarizes key organisms involved in the production of various siderophores and their respective biosynthetic genes .
Clinical Applications
Potential Therapeutic Uses:
The tumor-suppressive activity of this compound suggests its relevance in clinical settings, particularly in developing novel cancer therapies.
Research Insight:
The identification of ferrichrome as a bioactive compound with potential health benefits emphasizes the need for further exploration into its pharmacological properties and mechanisms of action .
Mechanism of Action
Ferrichrome A exerts its effects by chelating ferric iron through the oxygen atoms of its hydroxyl and acyl groups. This chelation forms a stable complex that can be transported into microbial cells. The molecular targets of this compound include ferric iron and the transport proteins that facilitate the uptake of the this compound-iron complex .
Comparison with Similar Compounds
Similar Compounds:
- Ferrichrome
- Ferrirubin
- Ferrirhodin
- Triacetylfusarinine C
Uniqueness: Ferrichrome A is unique among siderophores due to its specific structure and the particular fungi that produce it. While other siderophores like Ferrichrome, Ferrirubin, and Ferrirhodin also chelate iron, this compound’s distinct cyclic hexapeptide structure and its production by Ustilago maydis set it apart .
Biological Activity
Ferrichrome A, a member of the siderophore family, plays a crucial role in iron transport and metabolism in various microorganisms. This article delves into its biological activities, mechanisms of action, and the implications for health and disease, supported by research findings and case studies.
Overview of this compound
This compound is a fungal-type siderophore primarily produced by fungi and some bacteria. It is characterized by its ability to chelate iron (Fe³⁺), facilitating its uptake by cells in iron-limited environments. This property is essential for microbial growth and has implications for human health, particularly in the context of infections and cancer.
- Iron Uptake : this compound binds to iron with high affinity, which is critical for microbial survival in iron-depleted environments. In Pseudomonas aeruginosa, ferrichrome uptake occurs predominantly through the FiuA receptor, with TonB1 providing the necessary energy for transport across the membrane .
- Regulation of Metabolism : Research indicates that this compound influences metabolic pathways in yeast. For instance, it promotes adaptive growth in Schizosaccharomyces pombe under high ammonium conditions by enhancing amino acid uptake via specific transporters like Cat1 . This suggests that ferrichrome not only facilitates iron acquisition but also modulates nitrogen metabolism.
- Cancer Suppression : A notable study demonstrated that ferrichrome derived from Lactobacillus casei exhibits tumor-suppressive effects on colon cancer cells. Treatment with ferrichrome altered the expression of 265 mRNAs related to stress responses and apoptosis pathways, indicating its potential as a therapeutic agent against cancer progression .
Case Studies
- Fungal Growth Promotion : In a study involving S. pombe, this compound was shown to counteract the growth suppression caused by high ammonium concentrations. This effect was linked to enhanced leucine uptake, demonstrating its role in nutrient regulation under stress conditions .
- Antimicrobial Activity : this compound has been investigated for its antimicrobial properties. In Pseudomonas aeruginosa, it was found to compete with other siderophores for iron uptake, highlighting its significance in bacterial competition and survival strategies .
Table 1: Biological Effects of this compound
Table 2: Key Transporters Involved in this compound Uptake
Properties
IUPAC Name |
(Z)-5-[3-[5-[3-[[(E)-4-carboxy-3-methylbut-2-enoyl]-oxidoamino]propyl]-8-[3-[[(Z)-4-carboxy-3-methylbut-2-enoyl]-oxidoamino]propyl]-11,14-bis(hydroxymethyl)-3,6,9,12,15,18-hexaoxo-1,4,7,10,13,16-hexazacyclooctadec-2-yl]propyl-oxidoamino]-3-methyl-5-oxopent-3-enoic acid;iron(3+) | |
---|---|---|
Source | PubChem | |
URL | https://pubchem.ncbi.nlm.nih.gov | |
Description | Data deposited in or computed by PubChem | |
InChI |
InChI=1S/C41H58N9O20.Fe/c1-22(16-34(57)58)13-31(54)48(68)10-4-7-25-38(64)44-26(8-5-11-49(69)32(55)14-23(2)17-35(59)60)39(65)45-27(9-6-12-50(70)33(56)15-24(3)18-36(61)62)40(66)47-29(21-52)41(67)46-28(20-51)37(63)42-19-30(53)43-25;/h13-15,25-29,51-52H,4-12,16-21H2,1-3H3,(H,42,63)(H,43,53)(H,44,64)(H,45,65)(H,46,67)(H,47,66)(H,57,58)(H,59,60)(H,61,62);/q-3;+3/b22-13-,23-14+,24-15-; | |
Source | PubChem | |
URL | https://pubchem.ncbi.nlm.nih.gov | |
Description | Data deposited in or computed by PubChem | |
InChI Key |
GLLVIRNJIQOQJW-LDEMJYSLSA-N | |
Source | PubChem | |
URL | https://pubchem.ncbi.nlm.nih.gov | |
Description | Data deposited in or computed by PubChem | |
Canonical SMILES |
CC(=CC(=O)N(CCCC1C(=O)NC(C(=O)NC(C(=O)NC(C(=O)NC(C(=O)NCC(=O)N1)CO)CO)CCCN(C(=O)C=C(C)CC(=O)O)[O-])CCCN(C(=O)C=C(C)CC(=O)O)[O-])[O-])CC(=O)O.[Fe+3] | |
Source | PubChem | |
URL | https://pubchem.ncbi.nlm.nih.gov | |
Description | Data deposited in or computed by PubChem | |
Isomeric SMILES |
C/C(=C/C(=O)N(CCCC1C(=O)NC(C(=O)NC(C(=O)NC(C(=O)NC(C(=O)NCC(=O)N1)CO)CO)CCCN(C(=O)/C=C(/C)\CC(=O)O)[O-])CCCN(C(=O)/C=C(\C)/CC(=O)O)[O-])[O-])/CC(=O)O.[Fe+3] | |
Source | PubChem | |
URL | https://pubchem.ncbi.nlm.nih.gov | |
Description | Data deposited in or computed by PubChem | |
Molecular Formula |
C41H58FeN9O20 | |
Source | PubChem | |
URL | https://pubchem.ncbi.nlm.nih.gov | |
Description | Data deposited in or computed by PubChem | |
DSSTOX Substance ID |
DTXSID201336337 | |
Record name | Ferrichrome A | |
Source | EPA DSSTox | |
URL | https://comptox.epa.gov/dashboard/DTXSID201336337 | |
Description | DSSTox provides a high quality public chemistry resource for supporting improved predictive toxicology. | |
Molecular Weight |
1052.8 g/mol | |
Source | PubChem | |
URL | https://pubchem.ncbi.nlm.nih.gov | |
Description | Data deposited in or computed by PubChem | |
CAS No. |
15258-80-7 | |
Record name | Ferrichrome A | |
Source | ChemIDplus | |
URL | https://pubchem.ncbi.nlm.nih.gov/substance/?source=chemidplus&sourceid=0015258807 | |
Description | ChemIDplus is a free, web search system that provides access to the structure and nomenclature authority files used for the identification of chemical substances cited in National Library of Medicine (NLM) databases, including the TOXNET system. | |
Record name | Iron, [cyclo[glycyl-N5-[(2E)-4-carboxy-3-methyl-1-(oxo-.kappa.O)-2-buten-1-yl]-N5-(hydroxy-.kappa.O)-L-ornithyl-N5-[(2E)-4-carboxy-3-methyl-1-(oxo-.kappa.O)-2-buten-1-yl]-N5-(hydroxy-.kappa.O)-L-ornithyl-N5-[(2E)-4-carboxy-3-methyl-1-(oxo-.kappa.O)-2-buten-1-yl]-N5-(hydroxy-.kappa.O)-L-ornithyl-L-seryl-L-seryl]ato(3-)]-, (OC-6-64)- | |
Source | EPA Chemicals under the TSCA | |
URL | https://www.epa.gov/chemicals-under-tsca | |
Description | EPA Chemicals under the Toxic Substances Control Act (TSCA) collection contains information on chemicals and their regulations under TSCA, including non-confidential content from the TSCA Chemical Substance Inventory and Chemical Data Reporting. | |
Record name | Ferrichrome A | |
Source | EPA DSSTox | |
URL | https://comptox.epa.gov/dashboard/DTXSID201336337 | |
Description | DSSTox provides a high quality public chemistry resource for supporting improved predictive toxicology. | |
Record name | Ferrichrome A | |
Source | European Chemicals Agency (ECHA) | |
URL | https://echa.europa.eu/substance-information/-/substanceinfo/100.035.713 | |
Description | The European Chemicals Agency (ECHA) is an agency of the European Union which is the driving force among regulatory authorities in implementing the EU's groundbreaking chemicals legislation for the benefit of human health and the environment as well as for innovation and competitiveness. | |
Explanation | Use of the information, documents and data from the ECHA website is subject to the terms and conditions of this Legal Notice, and subject to other binding limitations provided for under applicable law, the information, documents and data made available on the ECHA website may be reproduced, distributed and/or used, totally or in part, for non-commercial purposes provided that ECHA is acknowledged as the source: "Source: European Chemicals Agency, http://echa.europa.eu/". Such acknowledgement must be included in each copy of the material. ECHA permits and encourages organisations and individuals to create links to the ECHA website under the following cumulative conditions: Links can only be made to webpages that provide a link to the Legal Notice page. | |
Retrosynthesis Analysis
AI-Powered Synthesis Planning: Our tool employs the Template_relevance Pistachio, Template_relevance Bkms_metabolic, Template_relevance Pistachio_ringbreaker, Template_relevance Reaxys, Template_relevance Reaxys_biocatalysis model, leveraging a vast database of chemical reactions to predict feasible synthetic routes.
One-Step Synthesis Focus: Specifically designed for one-step synthesis, it provides concise and direct routes for your target compounds, streamlining the synthesis process.
Accurate Predictions: Utilizing the extensive PISTACHIO, BKMS_METABOLIC, PISTACHIO_RINGBREAKER, REAXYS, REAXYS_BIOCATALYSIS database, our tool offers high-accuracy predictions, reflecting the latest in chemical research and data.
Strategy Settings
Precursor scoring | Relevance Heuristic |
---|---|
Min. plausibility | 0.01 |
Model | Template_relevance |
Template Set | Pistachio/Bkms_metabolic/Pistachio_ringbreaker/Reaxys/Reaxys_biocatalysis |
Top-N result to add to graph | 6 |
Feasible Synthetic Routes
Disclaimer and Information on In-Vitro Research Products
Please be aware that all articles and product information presented on BenchChem are intended solely for informational purposes. The products available for purchase on BenchChem are specifically designed for in-vitro studies, which are conducted outside of living organisms. In-vitro studies, derived from the Latin term "in glass," involve experiments performed in controlled laboratory settings using cells or tissues. It is important to note that these products are not categorized as medicines or drugs, and they have not received approval from the FDA for the prevention, treatment, or cure of any medical condition, ailment, or disease. We must emphasize that any form of bodily introduction of these products into humans or animals is strictly prohibited by law. It is essential to adhere to these guidelines to ensure compliance with legal and ethical standards in research and experimentation.