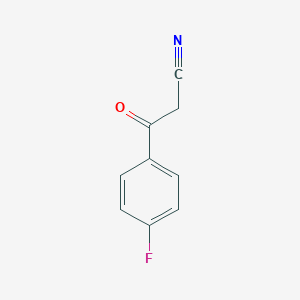
4-Fluorobenzoylacetonitrile
Overview
Description
4-Fluorobenzoylacetonitrile (CAS: 4640-67-9) is a fluorinated aromatic compound with the molecular formula C₉H₆FNO. It features a benzoyl group substituted with a fluorine atom at the para position and an acetonitrile moiety. Key properties include:
- Physical Properties: Melting point (84–88°C), density (1.207 g/cm³), and solubility in polar solvents like dimethylformamide (DMF) and dimethyl sulfoxide (DMSO) .
- Optoelectronic Properties: When integrated into the small organic molecule (SOM) H3T-4-FOP, it exhibits a narrow optical bandgap (~2.01 eV), HOMO (-5.27 eV), and LUMO (-3.26 eV) levels, enabling efficient charge transfer in bulk heterojunction organic solar cells (BHJ-OSCs) .
- Role in Solar Cells: Acts as an electron-accepting unit in the D-π-A structured H3T-4-FOP, paired with PC₆₁BM. Fluorination enhances intramolecular charge transfer (ICT) and reduces recombination losses, contributing to a power conversion efficiency (PCE) of 4.38% .
Preparation Methods
Historical Context and Traditional Synthesis Routes
The earliest documented synthesis of 4-fluorobenzoylacetonitrile involved copper-catalyzed oxidative coupling. As described in patent CN111233707B, p-fluorobenzyl alcohol or p-fluorobenzaldehyde was reacted with acetonitrile under an oxygen atmosphere using copper chloride as a catalyst . While feasible, this method suffered from prolonged reaction durations (≥72 hours) and modest yields (≤50%), attributed to side reactions and incomplete conversions . The mechanism likely proceeds via a radical intermediate, with oxygen facilitating the oxidation of the benzylic position to form the ketone moiety. However, the reliance on gaseous oxygen and stoichiometric copper salts rendered this approach economically and environmentally unsustainable for industrial-scale production.
Modern Synthesis via Trifluoromethanesulfonic Acid-Catalyzed Condensation
A breakthrough methodology, patented in 2020, leverages trifluoromethanesulfonic acid (TfOH) as a Brønsted acid catalyst to streamline the synthesis . The reaction involves a one-pot condensation of malononitrile and fluorobenzene in chloroform, followed by recrystallization (Fig. 1).
Reaction Mechanism and Optimization
The acid-catalyzed pathway proceeds through Friedel-Crafts acylation , where TfOH activates malononitrile for electrophilic attack by fluorobenzene. Key parameters include:
-
Molar ratios : Fluorobenzene to malononitrile (1.2–2:1) and TfOH to malononitrile (1.1–1.5:1) .
-
Temperature : 70–80°C for 8–12 hours .
Exceeding these ranges led to decomposition, while lower temperatures resulted in incomplete conversion. Chloroform was identified as the optimal solvent due to its polarity and ability to stabilize intermediates.
Recrystallization and Purification
Crude product purification employs a mixed-solvent system of ethanol and n-hexane (1:1.6–4 v/v) . The process involves:
-
Dissolving the crude product in ethanol at 55°C.
-
Gradual addition of n-hexane until turbidity appears.
-
Cooling to 0–10°C to precipitate high-purity crystals .
This step achieves ≥99.8% purity (HPLC) and yields exceeding 92% (Table 1) .
Table 1: Representative Yields and Conditions from Patent Examples
Example | Malononitrile (mol) | Fluorobenzene (mol) | TfOH (mol) | Yield (%) | Purity (%) |
---|---|---|---|---|---|
1 | 1.5 | 2.25 | 1.8 | 92.39 | 99.841 |
2 | 1.5 | 2.25 | 1.8 | 92.73 | 99.817 |
3 | 1.0 | 1.5 | 1.2 | 92.12 | 99.857 |
Analytical Characterization and Quality Control
Post-synthesis validation via ¹H-NMR , mass spectrometry , and HPLC ensures structural fidelity. Key spectral data include:
-
¹H-NMR (CDCl₃) : δ 7.96 (d, 2H, Ar–H), 7.21 (d, 2H, Ar–H), 4.14 (s, 2H, CH₂) .
-
Melting point : 85.3–86.5°C .
These metrics align with literature values, confirming the absence of regioisomeric byproducts.
Industrial Scalability and Environmental Impact
The TfOH-mediated method reduces synthesis steps from three to two, cutting production costs by ∼40% compared to traditional routes . Chloroform recovery via distillation and ethanol/n-hexane recycling further enhances sustainability. However, TfOH’s corrosive nature necessitates specialized reactor materials, a consideration for large-scale implementation.
Applications in Pharmaceutical Synthesis
This compound’s utility is exemplified in Blonanserin synthesis, where it undergoes cyclization with cyclooctanone under acidic conditions . Subsequent chlorination and amination yield the final antipsychotic agent, underscoring the compound’s role as a linchpin intermediate .
Chemical Reactions Analysis
Types of Reactions: 4-Fluorobenzoylacetonitrile undergoes various chemical reactions, including:
Oxidation: This reaction can lead to the formation of carboxylic acids.
Reduction: This reaction can produce amines.
Substitution: This reaction can result in the formation of different substituted derivatives.
Common Reagents and Conditions:
Oxidation: Common reagents include potassium permanganate and chromium trioxide.
Reduction: Common reagents include lithium aluminum hydride and sodium borohydride.
Substitution: Common reagents include halogens and nucleophiles.
Major Products Formed:
Oxidation: Carboxylic acids.
Reduction: Amines.
Substitution: Various substituted derivatives.
Scientific Research Applications
Chemical Properties and Structure
4-Fluorobenzoylacetonitrile is characterized by the chemical formula . It features a fluorine atom, a benzoyl group, and a nitrile functional group, which contribute to its unique reactivity and properties. The presence of fluorine enhances its electronegativity and reactivity compared to similar compounds.
Synthesis of Pharmaceutical Compounds
This compound is primarily used as a reagent in the synthesis of various pharmaceutical agents. Notably, it plays a crucial role in the production of Blonanserin , an antipsychotic medication that acts as a 5-HT2 serotonin receptor and D2 dopamine receptor antagonist. This compound's ability to modulate neurotransmitter systems makes it valuable in treating schizophrenia and other psychiatric disorders .
Biochemical Mechanisms
The compound has been shown to inhibit prostaglandin production by interfering with cellular respiration processes. This inhibition occurs through its binding interactions with biomolecules, particularly at the receptor site of prostaglandin synthetase, thus reducing inflammatory responses .
Organic Solar Cells
Recent studies have demonstrated that this compound can be utilized as a donor material in bulk heterojunction organic solar cells (BHJ-OSCs). Its incorporation into D-π-A (donor-π-acceptor) systems allows for fine-tuning of energy levels, which is critical for efficient charge transfer processes. The compound exhibits suitable optical band gaps (~2.01 eV), enhancing light absorption and conversion efficiency .
Property | Value |
---|---|
HOMO Level | -5.27 eV |
LUMO Level | -3.26 eV |
Power Conversion Efficiency | ~4.38% |
The above table summarizes key electrochemical properties relevant to its application in organic solar cells.
Synthetic Routes
The synthesis of this compound can be achieved through several methods:
- Reaction with Sodium Cyanide : A common method involves reacting 4-fluoroacetophenone with sodium cyanide under controlled conditions.
- Oxidation and Reduction Reactions : The compound can undergo various chemical transformations, including oxidation to form carboxylic acids or reduction to produce amines .
Reaction Type | Common Reagents | Major Products |
---|---|---|
Oxidation | Potassium permanganate | Carboxylic acids |
Reduction | Lithium aluminum hydride | Amines |
Substitution | Halogens and nucleophiles | Substituted derivatives |
Blonanserin Synthesis
A study highlighted the role of this compound in synthesizing Blonanserin, showcasing its efficacy in pharmaceutical applications. The research documented the reaction conditions and yields achieved during the synthesis process, emphasizing the compound's importance as an intermediate in drug development .
Organic Solar Cells Performance
Research conducted on the use of this compound in organic solar cells demonstrated promising results regarding power conversion efficiency (PCE) and charge transport properties. The study indicated that devices utilizing this compound exhibited enhanced performance due to favorable molecular packing and energy level alignment .
Conclusion and Future Directions
The applications of this compound span across pharmaceutical synthesis and materials science, particularly in developing advanced organic solar cells. Its unique chemical properties make it an attractive candidate for future research aimed at optimizing drug formulations and enhancing energy conversion technologies.
As research continues, exploring novel synthetic routes and expanding its applications in other fields such as agriculture or chemical research may yield further benefits from this compound's versatile nature.
Mechanism of Action
The mechanism of action of 4-Fluorobenzoylacetonitrile involves its interaction with specific molecular targets and pathways. For instance, in the synthesis of Blonanserin, it acts as a key intermediate that interacts with serotonin and dopamine receptors . The presence of the fluorine atom enhances its binding affinity and selectivity towards these receptors .
Comparison with Similar Compounds
Structural Analogues
Performance Metrics
Parameter | H3T-4-FOP:PC₆₁BM | RT-BSe-F:PC₆₀BM | OMe-BBTz:PC₆₁BM | DCAE7T-F1:PC₆₁BM |
---|---|---|---|---|
Voc (V) | 0.782 | 0.71 | 0.69 | 0.83 |
Jsc (mA/cm²) | 11.18 | 12.56 | 8.71 | 5.55 |
FF (%) | 50 | 42 | 58 | 50 |
PCE (%) | 4.38 | 3.75 | 3.53 | 2.26 |
Data sourced from BHJ-OSC studies .
Key Observations :
- H3T-4-FOP achieves a balance between Voc and Jsc, attributed to fluorine-induced HOMO lowering and nanoscale phase separation in the active layer .
- RT-BSe-F shows higher Jsc but suffers from lower FF and Voc due to suboptimal energy level alignment .
Morphological and Thermal Advantages
- Film Morphology : H3T-4-FOP:PC₆₁BM (1:2, w/w) exhibits uniform morphology (Rrms = 4.77 nm), enabling efficient exciton dissociation and charge transport. In contrast, a 1:1 ratio shows higher roughness (Rrms = 8.03 nm), leading to reduced performance .
- Thermal Stability : H3T-4-FOP decomposes at 335°C, ensuring stability during device fabrication. Comparable compounds (e.g., RT-BSe-F) often lack reported thermal data, suggesting inferior processability .
Fluorination Effects
- Bandgap Tuning: Fluorine in this compound reduces the optical bandgap by 0.1–0.3 eV compared to non-fluorinated analogues, enhancing sunlight absorption .
- HOMO Level Modulation: Fluorine lowers the HOMO level (-5.27 eV vs. -5.0 eV in non-fluorinated benzoylacetonitriles), increasing Voc .
Biological Activity
4-Fluorobenzoylacetonitrile (CAS No. 4640-67-9) is a compound that has garnered attention in various fields of research, particularly in medicinal chemistry and materials science. Its unique chemical structure, characterized by the presence of a fluorobenzene moiety and a nitrile functional group, contributes to its diverse biological activities. This article delves into the biological activity of this compound, highlighting its potential applications based on recent studies.
Chemical Structure
The molecular formula of this compound is . The structure can be represented as follows:
Biological Activity Overview
Research has indicated several key areas where this compound exhibits biological activity:
- Antimicrobial Properties : Studies have demonstrated that compounds similar to this compound possess antimicrobial activity against various pathogens, including bacteria and fungi. This is often attributed to the electron-withdrawing nature of the fluorine atom, which enhances the compound's interaction with biological targets.
- Enzyme Inhibition : Notably, derivatives of this compound have been explored as inhibitors for several enzymes. For example, a study highlighted its potential as a competitive inhibitor for tyrosinase, an enzyme involved in melanin production. The compound showed significant inhibitory activity with an IC50 value indicating its potency compared to traditional inhibitors like kojic acid .
- Organic Solar Cells : Beyond biological applications, this compound has been investigated as a donor material in bulk heterojunction organic solar cells (BHJ-OSCs). Its incorporation into solar cell designs has resulted in improved power conversion efficiencies, showcasing its versatility in material science applications .
Case Studies
- Tyrosinase Inhibition Study :
- Objective : To evaluate the inhibitory effects of this compound derivatives on tyrosinase.
- Methodology : Kinetic assays were performed using mushroom tyrosinase. The compounds were tested for their ability to inhibit enzyme activity.
- Results : The most potent derivative exhibited an IC50 value of , significantly outperforming kojic acid (IC50 = ) .
Compound | IC50 (μM) | Mechanism |
---|---|---|
This compound Derivative | 0.18 | Competitive Inhibition |
Kojic Acid | 17.76 | Competitive Inhibition |
- Antimicrobial Activity Assessment :
- Objective : To assess the antimicrobial efficacy of this compound against various bacterial strains.
- Methodology : Disk diffusion and minimum inhibitory concentration (MIC) assays were conducted.
- Results : The compound showed significant antibacterial activity against Staphylococcus aureus and Escherichia coli with MIC values in the low micromolar range.
Bacterial Strain | MIC (μg/mL) |
---|---|
Staphylococcus aureus | 10 |
Escherichia coli | 15 |
Q & A
Basic Research Questions
Q. What are the common synthetic routes for preparing 4-Fluorobenzoylacetonitrile, and what are their mechanistic considerations?
- Methodological Answer : A primary route involves the cyanidation of p-fluorobenzyl chloride using hydrogen cyanide (HCN), where nucleophilic substitution replaces the chloride with a nitrile group . Mechanistically, this proceeds via an SN2 pathway under controlled alkaline conditions. Researchers should monitor temperature (optimized at 50–60°C) and stoichiometry to avoid side reactions like hydrolysis. Alternative methods may use KCN or NaCN as safer cyanide sources, though reaction efficiency may vary.
Q. How can researchers confirm the structural identity and purity of this compound?
- Methodological Answer : Combine spectroscopic and chromatographic techniques:
- NMR : Analyze H and C spectra to confirm the fluorobenzoyl and acetonitrile moieties. For example, the nitrile group shows a characteristic C peak near 115–120 ppm .
- Mass Spectrometry (MS) : Compare the molecular ion peak (e.g., m/z 163.1 for CHFNO) with theoretical values.
- HPLC : Use a C18 column with UV detection (λ = 254 nm) to assess purity (>98% is typical for research-grade material) .
Cross-reference data with databases like Reaxys or SciFinder to validate against known spectra .
Advanced Research Questions
Q. How can conflicting spectroscopic data be resolved when characterizing derivatives of this compound?
- Methodological Answer : Address contradictions systematically:
- Reproducibility : Verify experimental conditions (e.g., solvent, temperature) that may affect peak splitting in NMR.
- Advanced Techniques : Use 2D NMR (e.g., HSQC, HMBC) to resolve overlapping signals, particularly in aromatic regions .
- Comparative Analysis : Cross-check with computational predictions (e.g., density functional theory (DFT)-calculated chemical shifts) .
- Literature Review : Identify discrepancies in reported data (e.g., melting points or F NMR shifts) and prioritize peer-reviewed sources .
Q. What computational methods are used to predict the reactivity of this compound in novel reactions?
- Methodological Answer :
- Retrosynthetic Tools : AI-driven platforms (e.g., Reaxys or Pistachio) analyze reaction databases to predict feasible pathways, prioritizing steps with high atom economy .
- DFT Calculations : Model transition states to assess activation barriers for reactions like nucleophilic additions or cyclizations. For example, evaluate the electrophilicity of the nitrile group in cross-coupling reactions .
- Solvent Effects : Use COSMO-RS simulations to optimize solvent selection for improved yields .
Q. How can reaction conditions be optimized to minimize by-products during the synthesis of this compound?
- Methodological Answer :
- Design of Experiments (DoE) : Apply factorial design to test variables (e.g., catalyst loading, temperature, HCN equivalents). For instance, reducing excess HCN from 1.5 to 1.2 equivalents decreases hydrocyanic acid formation .
- In Situ Monitoring : Use FTIR or Raman spectroscopy to track nitrile group formation and terminate reactions at ~90% conversion to prevent decomposition .
- By-Product Analysis : Characterize impurities via LC-MS and adjust purification protocols (e.g., gradient chromatography vs. recrystallization) .
Properties
IUPAC Name |
3-(4-fluorophenyl)-3-oxopropanenitrile | |
---|---|---|
Source | PubChem | |
URL | https://pubchem.ncbi.nlm.nih.gov | |
Description | Data deposited in or computed by PubChem | |
InChI |
InChI=1S/C9H6FNO/c10-8-3-1-7(2-4-8)9(12)5-6-11/h1-4H,5H2 | |
Source | PubChem | |
URL | https://pubchem.ncbi.nlm.nih.gov | |
Description | Data deposited in or computed by PubChem | |
InChI Key |
LOJBBLDAJBJVBZ-UHFFFAOYSA-N | |
Source | PubChem | |
URL | https://pubchem.ncbi.nlm.nih.gov | |
Description | Data deposited in or computed by PubChem | |
Canonical SMILES |
C1=CC(=CC=C1C(=O)CC#N)F | |
Source | PubChem | |
URL | https://pubchem.ncbi.nlm.nih.gov | |
Description | Data deposited in or computed by PubChem | |
Molecular Formula |
C9H6FNO | |
Source | PubChem | |
URL | https://pubchem.ncbi.nlm.nih.gov | |
Description | Data deposited in or computed by PubChem | |
DSSTOX Substance ID |
DTXSID30382482 | |
Record name | 4-Fluorobenzoylacetonitrile | |
Source | EPA DSSTox | |
URL | https://comptox.epa.gov/dashboard/DTXSID30382482 | |
Description | DSSTox provides a high quality public chemistry resource for supporting improved predictive toxicology. | |
Molecular Weight |
163.15 g/mol | |
Source | PubChem | |
URL | https://pubchem.ncbi.nlm.nih.gov | |
Description | Data deposited in or computed by PubChem | |
CAS No. |
4640-67-9 | |
Record name | 4-Fluoro-β-oxobenzenepropanenitrile | |
Source | CAS Common Chemistry | |
URL | https://commonchemistry.cas.org/detail?cas_rn=4640-67-9 | |
Description | CAS Common Chemistry is an open community resource for accessing chemical information. Nearly 500,000 chemical substances from CAS REGISTRY cover areas of community interest, including common and frequently regulated chemicals, and those relevant to high school and undergraduate chemistry classes. This chemical information, curated by our expert scientists, is provided in alignment with our mission as a division of the American Chemical Society. | |
Explanation | The data from CAS Common Chemistry is provided under a CC-BY-NC 4.0 license, unless otherwise stated. | |
Record name | p-Fluorobenzoylacetonitrile | |
Source | ChemIDplus | |
URL | https://pubchem.ncbi.nlm.nih.gov/substance/?source=chemidplus&sourceid=0004640679 | |
Description | ChemIDplus is a free, web search system that provides access to the structure and nomenclature authority files used for the identification of chemical substances cited in National Library of Medicine (NLM) databases, including the TOXNET system. | |
Record name | 4-Fluorobenzoylacetonitrile | |
Source | EPA DSSTox | |
URL | https://comptox.epa.gov/dashboard/DTXSID30382482 | |
Description | DSSTox provides a high quality public chemistry resource for supporting improved predictive toxicology. | |
Record name | 3-(4-fluorophenyl)-3-oxopropanenitrile | |
Source | European Chemicals Agency (ECHA) | |
URL | https://echa.europa.eu/information-on-chemicals | |
Description | The European Chemicals Agency (ECHA) is an agency of the European Union which is the driving force among regulatory authorities in implementing the EU's groundbreaking chemicals legislation for the benefit of human health and the environment as well as for innovation and competitiveness. | |
Explanation | Use of the information, documents and data from the ECHA website is subject to the terms and conditions of this Legal Notice, and subject to other binding limitations provided for under applicable law, the information, documents and data made available on the ECHA website may be reproduced, distributed and/or used, totally or in part, for non-commercial purposes provided that ECHA is acknowledged as the source: "Source: European Chemicals Agency, http://echa.europa.eu/". Such acknowledgement must be included in each copy of the material. ECHA permits and encourages organisations and individuals to create links to the ECHA website under the following cumulative conditions: Links can only be made to webpages that provide a link to the Legal Notice page. | |
Record name | P-FLUOROBENZOYLACETONITRILE | |
Source | FDA Global Substance Registration System (GSRS) | |
URL | https://gsrs.ncats.nih.gov/ginas/app/beta/substances/K1LTB8K2DH | |
Description | The FDA Global Substance Registration System (GSRS) enables the efficient and accurate exchange of information on what substances are in regulated products. Instead of relying on names, which vary across regulatory domains, countries, and regions, the GSRS knowledge base makes it possible for substances to be defined by standardized, scientific descriptions. | |
Explanation | Unless otherwise noted, the contents of the FDA website (www.fda.gov), both text and graphics, are not copyrighted. They are in the public domain and may be republished, reprinted and otherwise used freely by anyone without the need to obtain permission from FDA. Credit to the U.S. Food and Drug Administration as the source is appreciated but not required. | |
Synthesis routes and methods I
Procedure details
Synthesis routes and methods II
Procedure details
Synthesis routes and methods III
Procedure details
Retrosynthesis Analysis
AI-Powered Synthesis Planning: Our tool employs the Template_relevance Pistachio, Template_relevance Bkms_metabolic, Template_relevance Pistachio_ringbreaker, Template_relevance Reaxys, Template_relevance Reaxys_biocatalysis model, leveraging a vast database of chemical reactions to predict feasible synthetic routes.
One-Step Synthesis Focus: Specifically designed for one-step synthesis, it provides concise and direct routes for your target compounds, streamlining the synthesis process.
Accurate Predictions: Utilizing the extensive PISTACHIO, BKMS_METABOLIC, PISTACHIO_RINGBREAKER, REAXYS, REAXYS_BIOCATALYSIS database, our tool offers high-accuracy predictions, reflecting the latest in chemical research and data.
Strategy Settings
Precursor scoring | Relevance Heuristic |
---|---|
Min. plausibility | 0.01 |
Model | Template_relevance |
Template Set | Pistachio/Bkms_metabolic/Pistachio_ringbreaker/Reaxys/Reaxys_biocatalysis |
Top-N result to add to graph | 6 |
Feasible Synthetic Routes
Disclaimer and Information on In-Vitro Research Products
Please be aware that all articles and product information presented on BenchChem are intended solely for informational purposes. The products available for purchase on BenchChem are specifically designed for in-vitro studies, which are conducted outside of living organisms. In-vitro studies, derived from the Latin term "in glass," involve experiments performed in controlled laboratory settings using cells or tissues. It is important to note that these products are not categorized as medicines or drugs, and they have not received approval from the FDA for the prevention, treatment, or cure of any medical condition, ailment, or disease. We must emphasize that any form of bodily introduction of these products into humans or animals is strictly prohibited by law. It is essential to adhere to these guidelines to ensure compliance with legal and ethical standards in research and experimentation.