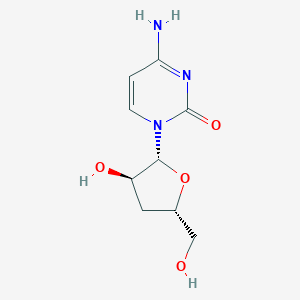
3'-Deoxycytidine
Overview
Description
3’-Deoxycytidine is a nucleoside analog that is structurally similar to cytidine but lacks a hydroxyl group at the 3’ position of the ribose sugar. This modification makes it a valuable compound in various biochemical and medical applications, particularly in the study of DNA synthesis and as a potential therapeutic agent.
Mechanism of Action
Target of Action
3’-Deoxycytidine primarily targets enzymes involved in DNA synthesis and modification. One of the key enzymes is deoxycytidine kinase (dCK) , which phosphorylates 3’-Deoxycytidine to form deoxycytidine monophosphate . Another target is cytidine deaminase (CDD) , which can inactivate deoxycytidine analogues .
Mode of Action
3’-Deoxycytidine, as a nucleoside analogue, is incorporated into the DNA during replication. It is phosphorylated by dCK into its active form, which can then be incorporated into the growing DNA strand . The presence of 3’-Deoxycytidine in the DNA can lead to premature chain termination, inhibiting DNA synthesis .
Biochemical Pathways
The metabolism of 3’-Deoxycytidine involves several pathways. It is phosphorylated by dCK, a rate-limiting step in its activation . It can also be inactivated by CDD or deoxycytidine monophosphate deaminase . Other metabolic pathways, such as those involving cytidine transporters, can also affect the activity of 3’-Deoxycytidine .
Pharmacokinetics
The pharmacokinetics of 3’-Deoxycytidine are influenced by its metabolism. It is rapidly inactivated by enzymes such as CDD, leading to a relatively short half-life . Coadministration with inhibitors of cdd, such as tetrahydrouridine, can increase its bioavailability .
Result of Action
The incorporation of 3’-Deoxycytidine into DNA leads to premature chain termination, inhibiting DNA synthesis and leading to cell cycle arrest, particularly in the S phase . This can potentially cause apoptosis of cells in the G1 and G2/M phases . It can also interfere with the methylation of certain genes, leading to increased expression of proteins that inhibit the transformation of certain diseases to more severe forms .
Action Environment
The action of 3’-Deoxycytidine can be influenced by various environmental factors. For instance, the presence of other nucleosides can compete with 3’-Deoxycytidine for the same metabolic pathways, affecting its activity . Additionally, the activity of enzymes such as CDD and dCK, which can vary between different cell types, can also influence the action of 3’-Deoxycytidine .
Biochemical Analysis
Biochemical Properties
3’-Deoxycytidine can be phosphorylated at C5’ of the deoxyribose by deoxycytidine kinase, converting it to deoxycytidine monophosphate (dCMP), a DNA precursor . This interaction with deoxycytidine kinase is crucial for its role in biochemical reactions. It also interacts with other enzymes and proteins, such as cytidine deaminase (CDD), which can inactivate it .
Cellular Effects
3’-Deoxycytidine has significant effects on various types of cells and cellular processes. It can slow the cell cycle by interfering with the methylation of the P15/INK4B gene, increasing the expression of P15/INK4B protein, which subdues the transformation of myelodysplastic syndromes (MDS) to leukemia . This indicates that 3’-Deoxycytidine influences cell function, including impact on cell signaling pathways, gene expression, and cellular metabolism.
Molecular Mechanism
The mechanism of action of 3’-Deoxycytidine involves its conversion to dCMP by deoxycytidine kinase, which is then further converted to dUMP and dTMP . This process involves binding interactions with the enzyme deoxycytidine kinase, leading to changes in gene expression and enzyme activation or inhibition.
Temporal Effects in Laboratory Settings
The effects of 3’-Deoxycytidine can change over time in laboratory settings. For instance, when 3’-Deoxycytidine was used in an engineered Escherichia coli strain, it showed high yield but also co-produced byproducts . This suggests that the stability, degradation, and long-term effects of 3’-Deoxycytidine on cellular function can vary in in vitro or in vivo studies.
Metabolic Pathways
3’-Deoxycytidine is involved in several metabolic pathways. It can be inactivated by cytidine deaminase (CDD), or by deoxycytidine monophosphate deaminase (dCMP deaminase). Additional metabolic pathways, such as phosphorylation, can substantially contribute to its (in)activation .
Transport and Distribution
The transport and distribution of 3’-Deoxycytidine within cells and tissues involve several factors. For instance, the aggregated impact of cytidine transporters, CDD, dCMP deaminase, and deoxycytidine kinase can influence 3’-Deoxycytidine metabolism .
Subcellular Localization
The subcellular localization of 3’-Deoxycytidine is primarily in the cytoplasm in several cell types, and the enzyme is more concentrated in the perinuclear and cellular membrane area . When 3’-Deoxycytidine is overexpressed in cells, it is mainly located in the nucleus . This suggests that 3’-Deoxycytidine may have targeting signals or post-translational modifications that direct it to specific compartments or organelles.
Preparation Methods
Synthetic Routes and Reaction Conditions: The synthesis of 3’-Deoxycytidine typically involves the selective removal of the hydroxyl group at the 3’ position of cytidine. One common method includes the use of cytidine deaminases and nucleoside phosphorylases in a multienzymatic cascade system . Another approach involves the transglycosylation reaction using 2-N-palmitoyl-guanine and 3’,5’-di-O-acetyl-4-N-benzoyl-2’-deoxycytidine as starting materials in the presence of a Lewis acid and silylating agent .
Industrial Production Methods: Industrial production of 3’-Deoxycytidine can be achieved through metabolic engineering of microbial strains. For instance, Escherichia coli can be genetically modified to enhance the production of deoxycytidine by deleting specific degradation enzymes and optimizing the biosynthetic pathway .
Chemical Reactions Analysis
Types of Reactions: 3’-Deoxycytidine undergoes various chemical reactions, including phosphorylation, deamination, and glycosylation. It can be phosphorylated at the 5’ position by deoxycytidine kinase to form deoxycytidine monophosphate (dCMP), which is a precursor for DNA synthesis .
Common Reagents and Conditions:
Phosphorylation: Deoxycytidine kinase and ATP.
Deamination: Cytidine deaminase.
Glycosylation: Lewis acids and silylating agents.
Major Products:
Phosphorylation: Deoxycytidine monophosphate (dCMP).
Deamination: Deoxyuridine.
Glycosylation: Various nucleoside analogs.
Scientific Research Applications
3’-Deoxycytidine has a wide range of applications in scientific research:
Chemistry: Used as a building block for the synthesis of nucleic acid derivatives and other nucleoside analogs.
Biology: Serves as a tool for studying DNA synthesis and repair mechanisms.
Medicine: Investigated for its potential as an antiviral and anticancer agent.
Comparison with Similar Compounds
3’-Deoxycytidine is similar to other nucleoside analogs such as:
2’-Deoxycytidine: Lacks a hydroxyl group at the 2’ position.
Zalcitabine (2’,3’-Dideoxycytidine): Lacks hydroxyl groups at both the 2’ and 3’ positions and is used as an antiretroviral drug.
Decitabine (5-Aza-2’-deoxycytidine): Contains an additional nitrogen atom in the pyrimidine ring and is used as an anticancer agent.
Uniqueness: The unique feature of 3’-Deoxycytidine is its selective removal of the 3’ hydroxyl group, which allows it to act as a chain terminator in DNA synthesis without the additional modifications found in other nucleoside analogs. This makes it a valuable tool in both research and therapeutic applications.
Properties
IUPAC Name |
4-amino-1-[(2R,3R,5S)-3-hydroxy-5-(hydroxymethyl)oxolan-2-yl]pyrimidin-2-one | |
---|---|---|
Source | PubChem | |
URL | https://pubchem.ncbi.nlm.nih.gov | |
Description | Data deposited in or computed by PubChem | |
InChI |
InChI=1S/C9H13N3O4/c10-7-1-2-12(9(15)11-7)8-6(14)3-5(4-13)16-8/h1-2,5-6,8,13-14H,3-4H2,(H2,10,11,15)/t5-,6+,8+/m0/s1 | |
Source | PubChem | |
URL | https://pubchem.ncbi.nlm.nih.gov | |
Description | Data deposited in or computed by PubChem | |
InChI Key |
ZHHOTKZTEUZTHX-SHYZEUOFSA-N | |
Source | PubChem | |
URL | https://pubchem.ncbi.nlm.nih.gov | |
Description | Data deposited in or computed by PubChem | |
Canonical SMILES |
C1C(OC(C1O)N2C=CC(=NC2=O)N)CO | |
Source | PubChem | |
URL | https://pubchem.ncbi.nlm.nih.gov | |
Description | Data deposited in or computed by PubChem | |
Isomeric SMILES |
C1[C@H](O[C@H]([C@@H]1O)N2C=CC(=NC2=O)N)CO | |
Source | PubChem | |
URL | https://pubchem.ncbi.nlm.nih.gov | |
Description | Data deposited in or computed by PubChem | |
Molecular Formula |
C9H13N3O4 | |
Source | PubChem | |
URL | https://pubchem.ncbi.nlm.nih.gov | |
Description | Data deposited in or computed by PubChem | |
DSSTOX Substance ID |
DTXSID80990793 | |
Record name | 1-(3-Deoxypentofuranosyl)-4-imino-1,4-dihydropyrimidin-2-ol | |
Source | EPA DSSTox | |
URL | https://comptox.epa.gov/dashboard/DTXSID80990793 | |
Description | DSSTox provides a high quality public chemistry resource for supporting improved predictive toxicology. | |
Molecular Weight |
227.22 g/mol | |
Source | PubChem | |
URL | https://pubchem.ncbi.nlm.nih.gov | |
Description | Data deposited in or computed by PubChem | |
CAS No. |
7057-33-2 | |
Record name | 3′-Deoxycytidine | |
Source | CAS Common Chemistry | |
URL | https://commonchemistry.cas.org/detail?cas_rn=7057-33-2 | |
Description | CAS Common Chemistry is an open community resource for accessing chemical information. Nearly 500,000 chemical substances from CAS REGISTRY cover areas of community interest, including common and frequently regulated chemicals, and those relevant to high school and undergraduate chemistry classes. This chemical information, curated by our expert scientists, is provided in alignment with our mission as a division of the American Chemical Society. | |
Explanation | The data from CAS Common Chemistry is provided under a CC-BY-NC 4.0 license, unless otherwise stated. | |
Record name | 3'-Deoxycytidine | |
Source | ChemIDplus | |
URL | https://pubchem.ncbi.nlm.nih.gov/substance/?source=chemidplus&sourceid=0007057332 | |
Description | ChemIDplus is a free, web search system that provides access to the structure and nomenclature authority files used for the identification of chemical substances cited in National Library of Medicine (NLM) databases, including the TOXNET system. | |
Record name | 1-(3-Deoxypentofuranosyl)-4-imino-1,4-dihydropyrimidin-2-ol | |
Source | EPA DSSTox | |
URL | https://comptox.epa.gov/dashboard/DTXSID80990793 | |
Description | DSSTox provides a high quality public chemistry resource for supporting improved predictive toxicology. | |
Retrosynthesis Analysis
AI-Powered Synthesis Planning: Our tool employs the Template_relevance Pistachio, Template_relevance Bkms_metabolic, Template_relevance Pistachio_ringbreaker, Template_relevance Reaxys, Template_relevance Reaxys_biocatalysis model, leveraging a vast database of chemical reactions to predict feasible synthetic routes.
One-Step Synthesis Focus: Specifically designed for one-step synthesis, it provides concise and direct routes for your target compounds, streamlining the synthesis process.
Accurate Predictions: Utilizing the extensive PISTACHIO, BKMS_METABOLIC, PISTACHIO_RINGBREAKER, REAXYS, REAXYS_BIOCATALYSIS database, our tool offers high-accuracy predictions, reflecting the latest in chemical research and data.
Strategy Settings
Precursor scoring | Relevance Heuristic |
---|---|
Min. plausibility | 0.01 |
Model | Template_relevance |
Template Set | Pistachio/Bkms_metabolic/Pistachio_ringbreaker/Reaxys/Reaxys_biocatalysis |
Top-N result to add to graph | 6 |
Feasible Synthetic Routes
A: 3'-Deoxycytidine, after being phosphorylated to its triphosphate form, primarily targets DNA polymerases and DNA primase. [, ] This chain-terminating nucleotide analog acts as a competitive inhibitor, mimicking natural substrates (dCTP for DNA polymerase and CTP/UTP for RNA polymerase). [, ] Incorporation of this compound triphosphate into DNA halts further DNA synthesis due to the absence of a 3'-hydroxyl group required for chain elongation. [, , ] This inhibition of DNA replication leads to cytotoxicity, particularly in rapidly dividing cells like cancer cells. [, ]
A:
- Spectroscopic Data:
ANone: While specific details about material compatibility aren't provided in the given papers, this compound is typically handled in laboratory settings using standard techniques for nucleoside analogs. Stability can vary depending on conditions:
- Transcription Start Site Mapping: The chain-terminating property of this compound triphosphate is exploited to specifically terminate RNA transcripts at cytosine residues, enabling the identification of transcription start sites. [, , ]
A: Yes, density functional theory (DFT) calculations have been used to study the conformational properties of this compound and its analogs. [] These calculations provided insights into the impact of structural modifications on the sugar puckering and intramolecular hydrogen bond network. [] Further research may involve QSAR modeling to predict the activity of novel analogs.
ANone: Several studies explore modifications of this compound and their impact:
- 3'-Hydroxyl Group: Essential for its activity. Replacing it with hydrogen (as in this compound) leads to loss of substrate activity for DNA polymerases. []
- Base Modifications:
- 5-Halogenation: 5-Bromo and 5-iodo derivatives of this compound demonstrated antiviral activity against Herpes Simplex Virus (HSV). []
- N4-Hydroxy: N4-Hydroxy-3'-deoxycytidine and its analogs were synthesized and tested against BVDV and HCV, but none showed superior activity to the parent compound. []
- Sugar Modifications:
- 2'-Modifications: 2'-O-acetyl, 2'-O-acetyl-5'-O-acetyl, and 2',5'-di-O-acetyl derivatives of 3'-deoxy-ara-C showed similar or slightly better anticancer activity compared to the parent compound. []
- Carbocyclic Analogs: Carbocyclic analogs of this compound exhibited varying degrees of antiviral activity against HSV and influenza virus, with carbodine showing promising activity against influenza. [, ]
ANone: While detailed formulation strategies for this compound are not discussed in the provided papers, information on its stability and potential approaches can be inferred:
A: The studies don't extensively cover this compound resistance, but one paper suggests that impaired cellular accumulation of the related compound 2',3'-dideoxy-3'-thiacytidine (3TC) may play a role in acquired resistance. [] This resistance was independent of multidrug resistant protein 4 (MRP4) but potentially linked to the upregulation of ATP-binding cassette C11 (ABCC11). [] Resistance mechanisms to nucleoside analogs often involve alterations in drug uptake, phosphorylation, or target enzyme activity.
ANone: The papers provided do not delve into detailed toxicology or long-term safety profiles.
ANone: Several papers mention in vitro studies:
- Cancer Cell Lines: 3'-Deoxy-ara-C, a close analog, displayed significant anticancer activity against various cancer cell lines (CCRF-CEM, L1210, P388, S-180) with varying ED50 values. []
- Viral Inhibition: this compound inhibited HCV-like RNA template replication in a cell-based assay. []
- Toad Bladder Model: While not a direct measure of this compound's efficacy, studies on toad bladder revealed that its action on sodium transport, influenced by aldosterone, was not sensitive to rRNA synthesis inhibition by this compound within the first 3 hours. []
ANone: Common analytical techniques mentioned include:
Disclaimer and Information on In-Vitro Research Products
Please be aware that all articles and product information presented on BenchChem are intended solely for informational purposes. The products available for purchase on BenchChem are specifically designed for in-vitro studies, which are conducted outside of living organisms. In-vitro studies, derived from the Latin term "in glass," involve experiments performed in controlled laboratory settings using cells or tissues. It is important to note that these products are not categorized as medicines or drugs, and they have not received approval from the FDA for the prevention, treatment, or cure of any medical condition, ailment, or disease. We must emphasize that any form of bodily introduction of these products into humans or animals is strictly prohibited by law. It is essential to adhere to these guidelines to ensure compliance with legal and ethical standards in research and experimentation.