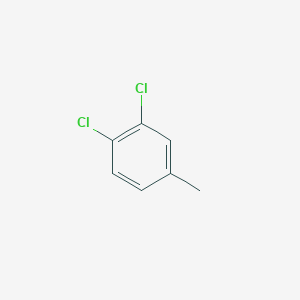
3,4-Dichlorotoluene
Overview
Description
3,4-Dichlorotoluene is an organic compound with the chemical formula CH₃C₆H₃Cl₂. It is a colorless liquid with a characteristic odor and is primarily used as an intermediate in the synthesis of various chemicals. This compound is part of the chlorotoluene family, where chlorine atoms are substituted on the benzene ring of toluene.
Scientific Research Applications
3,4-Dichlorotoluene has several applications in scientific research:
Chemistry: It is used as an intermediate in the synthesis of various organic compounds, including pharmaceuticals and agrochemicals.
Biology: The compound is used in studies involving the degradation of chlorinated aromatic compounds by microorganisms.
Medicine: It serves as a precursor in the synthesis of certain medicinal compounds.
Industry: It is employed in the production of dyes, pigments, and other specialty chemicals.
Safety and Hazards
Future Directions
Mechanism of Action
Target of Action
3,4-Dichlorotoluene (3,4-DCT) is an organic compound primarily used as a solvent and in organic synthesis . The primary targets of 3,4-DCT are enzymes involved in its metabolic degradation, such as the broad-spectrum chlorobenzene dioxygenase and chlorobenzene dihydrodiol dehydrogenase .
Mode of Action
The mode of action of 3,4-DCT involves its interaction with these enzymes. The compound is initially activated by dioxygenation, catalyzed by the chlorobenzene dioxygenase, followed by rearomatization catalyzed by chlorobenzene dihydrodiol dehydrogenase, resulting in the formation of 3,4-dichloro-6-methylcatechol .
Biochemical Pathways
The biochemical pathway of 3,4-DCT degradation involves the formation of dichloromethylcatechols as central intermediates . These intermediates are then channeled into a chlorocatechol ortho cleavage pathway involving a chlorocatechol 1,2-dioxygenase, chloromuconate cycloisomerase, and dienelactone hydrolase . Different metabolic routes are observed for the three dichloromethylcatechols analyzed .
Pharmacokinetics
It’s known that 3,4-dct is a volatile compound with a boiling point of 2005 °C , suggesting that it could be rapidly absorbed and distributed in the body following inhalation.
Result of Action
The result of the action of 3,4-DCT is its transformation into less harmful compounds through the aforementioned metabolic pathways . This process is crucial for the bioremediation of environments contaminated with chlorosubstituted toluenes and benzoates .
Action Environment
The action of 3,4-DCT is influenced by environmental factors. For instance, the presence of a catalyst like HZSM-5 can influence the isomerization of 2,5-dichlorotoluene to produce 2,4-dichlorotoluene . Additionally, the reaction temperature, the molar ratio of air/DCT, the molar ratio of NH3/DCT in the feed gas, and the space velocity can also affect the conversion, yield, and selectivity of the reaction .
Biochemical Analysis
Biochemical Properties
3,4-Dichlorotoluene is initially activated by dioxygenation, catalyzed by the broad spectrum chlorobenzene dioxygenase, followed by rearomatization catalyzed by chlorobenzene dihydrodiol dehydrogenase . This results in the formation of 3,4-dichloro-6-methylcatechol .
Molecular Mechanism
The molecular mechanism of this compound involves its conversion into 3,4-dichloro-6-methylcatechol via the action of chlorobenzene dioxygenase and chlorobenzene dihydrodiol dehydrogenase . This suggests that it may interact with these enzymes at the molecular level.
Metabolic Pathways
This compound is involved in the chlorotoluene degradation pathway, where it is converted into 3,4-dichloro-6-methylcatechol . This process involves the enzymes chlorobenzene dioxygenase and chlorobenzene dihydrodiol dehydrogenase .
Preparation Methods
Synthetic Routes and Reaction Conditions: 3,4-Dichlorotoluene can be synthesized through the chlorination of toluene. The process involves the reaction of toluene with chlorine gas in the presence of a catalyst such as ferric chloride (FeCl₃). The reaction is typically carried out at elevated temperatures to ensure the substitution of hydrogen atoms by chlorine atoms on the benzene ring.
Industrial Production Methods: In industrial settings, the production of this compound involves the controlled chlorination of toluene. The process parameters, such as temperature, pressure, and the concentration of chlorine gas, are carefully monitored to achieve the desired product with high purity. The reaction is usually conducted in a continuous flow reactor to optimize the yield and efficiency.
Chemical Reactions Analysis
Types of Reactions: 3,4-Dichlorotoluene undergoes various chemical reactions, including:
Oxidation: It can be oxidized to form 3,4-dichlorobenzaldehyde or 3,4-dichlorobenzoic acid using oxidizing agents such as potassium permanganate (KMnO₄) or chromium trioxide (CrO₃).
Reduction: The compound can be reduced to this compound using reducing agents like lithium aluminum hydride (LiAlH₄).
Substitution: It can undergo nucleophilic substitution reactions where the chlorine atoms are replaced by other functional groups. For example, reacting with sodium methoxide (NaOCH₃) can yield 3,4-dimethoxytoluene.
Common Reagents and Conditions:
Oxidation: Potassium permanganate in an acidic medium.
Reduction: Lithium aluminum hydride in anhydrous ether.
Substitution: Sodium methoxide in methanol.
Major Products Formed:
Oxidation: 3,4-Dichlorobenzaldehyde, 3,4-Dichlorobenzoic acid.
Reduction: this compound.
Substitution: 3,4-Dimethoxytoluene.
Comparison with Similar Compounds
- 2,4-Dichlorotoluene
- 2,5-Dichlorotoluene
- 2,6-Dichlorotoluene
- 3,5-Dichlorotoluene
Properties
IUPAC Name |
1,2-dichloro-4-methylbenzene | |
---|---|---|
Source | PubChem | |
URL | https://pubchem.ncbi.nlm.nih.gov | |
Description | Data deposited in or computed by PubChem | |
InChI |
InChI=1S/C7H6Cl2/c1-5-2-3-6(8)7(9)4-5/h2-4H,1H3 | |
Source | PubChem | |
URL | https://pubchem.ncbi.nlm.nih.gov | |
Description | Data deposited in or computed by PubChem | |
InChI Key |
WYUIWKFIFOJVKW-UHFFFAOYSA-N | |
Source | PubChem | |
URL | https://pubchem.ncbi.nlm.nih.gov | |
Description | Data deposited in or computed by PubChem | |
Canonical SMILES |
CC1=CC(=C(C=C1)Cl)Cl | |
Source | PubChem | |
URL | https://pubchem.ncbi.nlm.nih.gov | |
Description | Data deposited in or computed by PubChem | |
Molecular Formula |
C7H6Cl2 | |
Source | PubChem | |
URL | https://pubchem.ncbi.nlm.nih.gov | |
Description | Data deposited in or computed by PubChem | |
DSSTOX Substance ID |
DTXSID2021814 | |
Record name | 3,4-Dichlorotoluene | |
Source | EPA DSSTox | |
URL | https://comptox.epa.gov/dashboard/DTXSID2021814 | |
Description | DSSTox provides a high quality public chemistry resource for supporting improved predictive toxicology. | |
Molecular Weight |
161.03 g/mol | |
Source | PubChem | |
URL | https://pubchem.ncbi.nlm.nih.gov | |
Description | Data deposited in or computed by PubChem | |
CAS No. |
95-75-0 | |
Record name | 3,4-Dichlorotoluene | |
Source | CAS Common Chemistry | |
URL | https://commonchemistry.cas.org/detail?cas_rn=95-75-0 | |
Description | CAS Common Chemistry is an open community resource for accessing chemical information. Nearly 500,000 chemical substances from CAS REGISTRY cover areas of community interest, including common and frequently regulated chemicals, and those relevant to high school and undergraduate chemistry classes. This chemical information, curated by our expert scientists, is provided in alignment with our mission as a division of the American Chemical Society. | |
Explanation | The data from CAS Common Chemistry is provided under a CC-BY-NC 4.0 license, unless otherwise stated. | |
Record name | Benzene, 1,2-dichloro-4-methyl- | |
Source | ChemIDplus | |
URL | https://pubchem.ncbi.nlm.nih.gov/substance/?source=chemidplus&sourceid=0000095750 | |
Description | ChemIDplus is a free, web search system that provides access to the structure and nomenclature authority files used for the identification of chemical substances cited in National Library of Medicine (NLM) databases, including the TOXNET system. | |
Record name | 3,4-DICHLOROTOLUENE | |
Source | DTP/NCI | |
URL | https://dtp.cancer.gov/dtpstandard/servlet/dwindex?searchtype=NSC&outputformat=html&searchlist=8765 | |
Description | The NCI Development Therapeutics Program (DTP) provides services and resources to the academic and private-sector research communities worldwide to facilitate the discovery and development of new cancer therapeutic agents. | |
Explanation | Unless otherwise indicated, all text within NCI products is free of copyright and may be reused without our permission. Credit the National Cancer Institute as the source. | |
Record name | Benzene, 1,2-dichloro-4-methyl- | |
Source | EPA Chemicals under the TSCA | |
URL | https://www.epa.gov/chemicals-under-tsca | |
Description | EPA Chemicals under the Toxic Substances Control Act (TSCA) collection contains information on chemicals and their regulations under TSCA, including non-confidential content from the TSCA Chemical Substance Inventory and Chemical Data Reporting. | |
Record name | 3,4-Dichlorotoluene | |
Source | EPA DSSTox | |
URL | https://comptox.epa.gov/dashboard/DTXSID2021814 | |
Description | DSSTox provides a high quality public chemistry resource for supporting improved predictive toxicology. | |
Record name | 3,4-dichlorotoluene | |
Source | European Chemicals Agency (ECHA) | |
URL | https://echa.europa.eu/substance-information/-/substanceinfo/100.002.226 | |
Description | The European Chemicals Agency (ECHA) is an agency of the European Union which is the driving force among regulatory authorities in implementing the EU's groundbreaking chemicals legislation for the benefit of human health and the environment as well as for innovation and competitiveness. | |
Explanation | Use of the information, documents and data from the ECHA website is subject to the terms and conditions of this Legal Notice, and subject to other binding limitations provided for under applicable law, the information, documents and data made available on the ECHA website may be reproduced, distributed and/or used, totally or in part, for non-commercial purposes provided that ECHA is acknowledged as the source: "Source: European Chemicals Agency, http://echa.europa.eu/". Such acknowledgement must be included in each copy of the material. ECHA permits and encourages organisations and individuals to create links to the ECHA website under the following cumulative conditions: Links can only be made to webpages that provide a link to the Legal Notice page. | |
Retrosynthesis Analysis
AI-Powered Synthesis Planning: Our tool employs the Template_relevance Pistachio, Template_relevance Bkms_metabolic, Template_relevance Pistachio_ringbreaker, Template_relevance Reaxys, Template_relevance Reaxys_biocatalysis model, leveraging a vast database of chemical reactions to predict feasible synthetic routes.
One-Step Synthesis Focus: Specifically designed for one-step synthesis, it provides concise and direct routes for your target compounds, streamlining the synthesis process.
Accurate Predictions: Utilizing the extensive PISTACHIO, BKMS_METABOLIC, PISTACHIO_RINGBREAKER, REAXYS, REAXYS_BIOCATALYSIS database, our tool offers high-accuracy predictions, reflecting the latest in chemical research and data.
Strategy Settings
Precursor scoring | Relevance Heuristic |
---|---|
Min. plausibility | 0.01 |
Model | Template_relevance |
Template Set | Pistachio/Bkms_metabolic/Pistachio_ringbreaker/Reaxys/Reaxys_biocatalysis |
Top-N result to add to graph | 6 |
Feasible Synthetic Routes
Q1: What is the molecular formula and weight of 3,4-Dichlorotoluene?
A1: this compound has the molecular formula C₇H₆Cl₂ and a molecular weight of 161.03 g/mol. []
Q2: What are the main applications of this compound?
A2: this compound serves as a crucial starting material in the synthesis of various chemicals. One prominent application is its use in producing 3,4-dichlorobenzonitrile (DCBN), a valuable intermediate in the production of pesticides and pharmaceuticals. [, ] Additionally, it can be utilized to synthesize 3,4-dichlorobenzoic acid, another important chemical intermediate. [, ]
Q3: How is 3,4-dichlorobenzonitrile synthesized from this compound?
A3: 3,4-Dichlorobenzonitrile can be synthesized from this compound through a process called ammoxidation. This gas-phase reaction involves reacting this compound with ammonia and oxygen in the presence of a catalyst. Researchers have investigated different catalysts for this process, including DN120 [] and VPO/SiO2. []
Q4: What factors influence the yield of 3,4-dichlorobenzonitrile during ammoxidation?
A4: The yield of 3,4-dichlorobenzonitrile in the ammoxidation of this compound is influenced by several reaction parameters. These include:
- Reaction temperature: Studies have shown that the optimal reaction temperature is around 400 °C. []
- Molar ratio of reactants: The molar ratio of air to this compound and ammonia to this compound significantly impacts the yield. []
- Catalyst: The choice of catalyst and its properties play a crucial role in determining the reaction efficiency and product selectivity. [, ]
Q5: Can this compound be degraded by microorganisms?
A5: Yes, certain bacterial strains can utilize this compound as a growth substrate and degrade it. For instance, Ralstonia sp. strain PS12 can break down this compound via a metabolic pathway involving the enzyme tetrachlorobenzene dioxygenase (TecA). [, ]
Q6: What is the role of tetrachlorobenzene dioxygenase (TecA) in this compound degradation?
A6: TecA catalyzes the initial step in the aerobic biodegradation of this compound by Ralstonia sp. strain PS12. This enzyme introduces two oxygen atoms into the aromatic ring of this compound, forming a dihydrodihydroxy derivative. This intermediate is then further metabolized by downstream enzymes in the degradation pathway. [, , ]
Q7: Can the regioselectivity of TecA be modified?
A7: Yes, research suggests that the regioselectivity of TecA can be engineered to some extent. Studies have identified specific amino acid residues in the active site of TecA that influence the site of oxygenation on the aromatic ring of chlorinated toluenes. [] By introducing mutations at these positions, researchers have observed changes in the product spectrum, indicating altered regioselectivity.
Q8: What is the significance of accurate density measurements for this compound?
A8: Precise density measurements are crucial for various scientific and industrial applications of this compound. These include:
- Material characterization: Density is a fundamental physical property used to identify and characterize substances. []
- Thermodynamic calculations: Density data is essential for thermodynamic modeling and calculations related to fluid behavior. []
- Process engineering: Accurate density information is critical for designing and optimizing chemical processes involving this compound. []
Q9: What method has been employed for accurate density measurements of this compound?
A9: Researchers have utilized a magnetic suspension balance system to achieve highly accurate density measurements of this compound. This method involves measuring the buoyant force acting on a silicon sinker immersed in the liquid, enabling precise density determination. []
Q10: How does the dielectric relaxation process differ among isomeric dichlorotoluenes?
A10: Studies using dielectric spectroscopy have revealed differences in the molecular dynamics and dielectric relaxation processes among isomers of dichlorotoluene, including 2,6-, 2,4-, and this compound. These differences in relaxation behavior are attributed to variations in molecular shape, dipole moment, and intermolecular interactions arising from the different positions of chlorine atoms on the aromatic ring. [, ]
Q11: How does the chlorine substitution pattern influence the Penning ionization electron spectra (PIES) of dichlorotoluenes?
A11: The number and position of chlorine substituents significantly impact the PIES of dichlorotoluenes. Research has shown that the relative intensities of bands in the PIES are sensitive to the spatial distribution of molecular orbitals, which are affected by the electron-withdrawing nature and steric effects of chlorine atoms. []
Q12: How does the chlorination of para-chlorotoluene proceed kinetically?
A12: The catalytic chlorination of para-chlorotoluene, producing 2,4-dichlorotoluene and this compound, follows a first-order parallel reaction mechanism. This means that para-chlorotoluene reacts independently to form each product, with the rate of each reaction pathway determined by its specific rate constant. []
Q13: What is the role of the catalyst in the chlorination of para-chlorotoluene?
A13: Catalysts, such as SbCl₃, play a crucial role in facilitating the chlorination of para-chlorotoluene by lowering the activation energy of the reaction. This results in a significant increase in reaction rate, making the process more efficient. []
Q14: What is the influence of catalyst concentration on the chlorination reaction?
A14: Increasing the catalyst concentration generally leads to a faster reaction rate in the chlorination of para-chlorotoluene. This is because a higher catalyst concentration provides more active sites for the reaction to occur, enhancing the overall reaction rate. []
Q15: Have any new 1,2-dihydrophthalazines been synthesized using this compound as a starting material?
A15: Yes, researchers have successfully synthesized novel 6,7-dichloro-4-(4-aminophenyl)-2-(N-alkylcarbamoyl)-1,2-dihydrophthalazines starting from this compound. This synthetic route involves several steps, including the conversion of this compound to the corresponding ortho-formylbenzophenone derivative, followed by further chemical transformations to yield the desired 1,2-dihydrophthalazine derivatives. []
Disclaimer and Information on In-Vitro Research Products
Please be aware that all articles and product information presented on BenchChem are intended solely for informational purposes. The products available for purchase on BenchChem are specifically designed for in-vitro studies, which are conducted outside of living organisms. In-vitro studies, derived from the Latin term "in glass," involve experiments performed in controlled laboratory settings using cells or tissues. It is important to note that these products are not categorized as medicines or drugs, and they have not received approval from the FDA for the prevention, treatment, or cure of any medical condition, ailment, or disease. We must emphasize that any form of bodily introduction of these products into humans or animals is strictly prohibited by law. It is essential to adhere to these guidelines to ensure compliance with legal and ethical standards in research and experimentation.