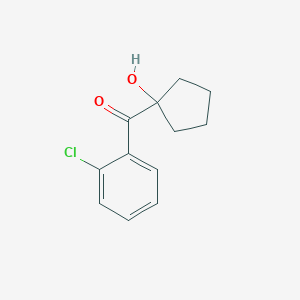
(2-Chlorophenyl) (1-hydroxycyclopentyl) ketone
Overview
Description
(2-Chlorophenyl) (1-hydroxycyclopentyl) ketone is an organic compound with the molecular formula C12H13ClO2 and a molecular weight of 224.68 g/mol . It is also known by other names such as (2-chlorophenyl) (1-hydroxycyclopentyl)methanone and 1-Hydroxycyclopentyl 2-chlorophenyl ketone . This compound is often used as an intermediate in the synthesis of various pharmaceuticals and research chemicals.
Mechanism of Action
Target of Action
Mode of Action
Biochemical Pathways
Result of Action
Preparation Methods
Synthetic Routes and Reaction Conditions
The synthesis of (2-Chlorophenyl) (1-hydroxycyclopentyl) ketone typically involves the Friedel-Crafts acylation reaction. This method uses (2-chlorophenyl) as an intermediate and involves the use of non-toxic materials and avoids corrosive acids. Enhanced by microwave heating, this method offers higher yields and cleaner products compared to conventional heating techniques.
Industrial Production Methods
In industrial settings, the production of this compound may involve large-scale Friedel-Crafts acylation reactions, optimized for higher efficiency and yield. The reaction conditions are carefully controlled to ensure the purity and quality of the final product.
Chemical Reactions Analysis
Types of Reactions
(2-Chlorophenyl) (1-hydroxycyclopentyl) ketone undergoes various chemical reactions, including:
Oxidation: This compound can be oxidized to form different products depending on the reagents and conditions used.
Reduction: Reduction reactions can convert the ketone group to an alcohol group.
Substitution: The chlorine atom in the phenyl ring can be substituted with other functional groups.
Common Reagents and Conditions
Oxidation: Common oxidizing agents include potassium permanganate (KMnO4) and chromium trioxide (CrO3).
Reduction: Reducing agents such as sodium borohydride (NaBH4) and lithium aluminum hydride (LiAlH4) are commonly used.
Substitution: Nucleophilic substitution reactions can be carried out using reagents like sodium methoxide (NaOCH3) or potassium tert-butoxide (KOtBu).
Major Products Formed
Oxidation: Products may include carboxylic acids or aldehydes.
Reduction: The major product is the corresponding alcohol.
Substitution: Products vary depending on the substituent introduced.
Scientific Research Applications
(2-Chlorophenyl) (1-hydroxycyclopentyl) ketone has several applications in scientific research:
Chemistry: Used as an intermediate in the synthesis of various organic compounds.
Biology: Studied for its potential biological activities and interactions with biological molecules.
Medicine: Investigated for its potential use in the development of new pharmaceuticals.
Industry: Utilized in the production of fine chemicals and as a reference standard in analytical chemistry.
Comparison with Similar Compounds
Similar Compounds
- (2-Chlorophenyl) (1-hydroxycyclopentyl)methanone
- 1-Hydroxycyclopentyl 2-chlorophenyl ketone
- Ketamine Impurity C
Comparison
Compared to similar compounds, (2-Chlorophenyl) (1-hydroxycyclopentyl) ketone is unique due to its specific molecular structure, which allows for distinct interactions with biological targets. Its synthesis methods and reaction conditions also offer advantages in terms of yield and purity.
Biological Activity
(2-Chlorophenyl) (1-hydroxycyclopentyl) ketone, with the molecular formula C12H13ClO2 and a molecular weight of 224.68 g/mol, is an organic compound recognized for its potential biological activities. This compound serves as an intermediate in the synthesis of various psychoactive substances, notably ketamine, which has garnered attention for its anesthetic and dissociative properties. Understanding the biological activity of this compound is crucial in both pharmacological research and forensic science.
The synthesis of this compound typically involves a Friedel-Crafts acylation reaction , utilizing non-toxic materials to enhance yield and purity. This method has been optimized using microwave heating to achieve higher efficiency compared to traditional methods.
The biological activity of this compound is primarily linked to its role as a precursor in the synthesis of ketamine. Ketamine acts as a dissociative anesthetic , affecting neurotransmitter systems in the brain, particularly by antagonizing the NMDA receptor. This interaction leads to its anesthetic effects and potential therapeutic applications in treating depression and chronic pain.
Cellular Effects
Research indicates that this compound exhibits significant cellular effects, primarily through its conversion to ketamine. In laboratory settings, this compound has been shown to influence various biochemical pathways, including those involved in pain perception and mood regulation .
Case Studies
- Synthesis and Analysis : A study focused on a chemical company's importation of precursors for illicit drug manufacturing highlighted the identification of this compound during forensic analysis. Techniques such as gas chromatography-mass spectrometry were employed to confirm its presence alongside ketamine, emphasizing its relevance in illicit drug synthesis .
- Biological Activity Assessment : A comprehensive review indicated that compounds similar to this compound exhibit varying degrees of biological activity. Notably, compounds with structural similarities were assessed for their dissociative properties, reinforcing the significance of this compound in the context of psychoactive substances.
Comparative Analysis with Similar Compounds
Compound Name | Biological Activity | Notes |
---|---|---|
This compound | Significant as a precursor for ketamine | Dissociative anesthetic properties |
Ketamine | Potent NMDA receptor antagonist | Used in anesthesia and depression treatment |
1-Hydroxycyclopentyl-(o-chlorophenyl) ketone | Similar metabolic pathways as ketamine | Investigated for synthetic routes |
Properties
IUPAC Name |
(2-chlorophenyl)-(1-hydroxycyclopentyl)methanone | |
---|---|---|
Source | PubChem | |
URL | https://pubchem.ncbi.nlm.nih.gov | |
Description | Data deposited in or computed by PubChem | |
InChI |
InChI=1S/C12H13ClO2/c13-10-6-2-1-5-9(10)11(14)12(15)7-3-4-8-12/h1-2,5-6,15H,3-4,7-8H2 | |
Source | PubChem | |
URL | https://pubchem.ncbi.nlm.nih.gov | |
Description | Data deposited in or computed by PubChem | |
InChI Key |
OVTPOHDQDJTAEF-UHFFFAOYSA-N | |
Source | PubChem | |
URL | https://pubchem.ncbi.nlm.nih.gov | |
Description | Data deposited in or computed by PubChem | |
Canonical SMILES |
C1CCC(C1)(C(=O)C2=CC=CC=C2Cl)O | |
Source | PubChem | |
URL | https://pubchem.ncbi.nlm.nih.gov | |
Description | Data deposited in or computed by PubChem | |
Molecular Formula |
C12H13ClO2 | |
Source | PubChem | |
URL | https://pubchem.ncbi.nlm.nih.gov | |
Description | Data deposited in or computed by PubChem | |
DSSTOX Substance ID |
DTXSID80238243 | |
Record name | (2-Chlorophenyl) (1-hydroxycyclopentyl) ketone | |
Source | EPA DSSTox | |
URL | https://comptox.epa.gov/dashboard/DTXSID80238243 | |
Description | DSSTox provides a high quality public chemistry resource for supporting improved predictive toxicology. | |
Molecular Weight |
224.68 g/mol | |
Source | PubChem | |
URL | https://pubchem.ncbi.nlm.nih.gov | |
Description | Data deposited in or computed by PubChem | |
CAS No. |
90717-17-2 | |
Record name | (2-Chlorophenyl)(1-hydroxycyclopentyl)methanone | |
Source | CAS Common Chemistry | |
URL | https://commonchemistry.cas.org/detail?cas_rn=90717-17-2 | |
Description | CAS Common Chemistry is an open community resource for accessing chemical information. Nearly 500,000 chemical substances from CAS REGISTRY cover areas of community interest, including common and frequently regulated chemicals, and those relevant to high school and undergraduate chemistry classes. This chemical information, curated by our expert scientists, is provided in alignment with our mission as a division of the American Chemical Society. | |
Explanation | The data from CAS Common Chemistry is provided under a CC-BY-NC 4.0 license, unless otherwise stated. | |
Record name | (2-Chlorophenyl) (1-hydroxycyclopentyl) ketone | |
Source | ChemIDplus | |
URL | https://pubchem.ncbi.nlm.nih.gov/substance/?source=chemidplus&sourceid=0090717172 | |
Description | ChemIDplus is a free, web search system that provides access to the structure and nomenclature authority files used for the identification of chemical substances cited in National Library of Medicine (NLM) databases, including the TOXNET system. | |
Record name | (2-Chlorophenyl) (1-hydroxycyclopentyl) ketone | |
Source | EPA DSSTox | |
URL | https://comptox.epa.gov/dashboard/DTXSID80238243 | |
Description | DSSTox provides a high quality public chemistry resource for supporting improved predictive toxicology. | |
Record name | (2-chlorophenyl) (1-hydroxycyclopentyl) ketone | |
Source | European Chemicals Agency (ECHA) | |
URL | https://echa.europa.eu/substance-information/-/substanceinfo/100.084.220 | |
Description | The European Chemicals Agency (ECHA) is an agency of the European Union which is the driving force among regulatory authorities in implementing the EU's groundbreaking chemicals legislation for the benefit of human health and the environment as well as for innovation and competitiveness. | |
Explanation | Use of the information, documents and data from the ECHA website is subject to the terms and conditions of this Legal Notice, and subject to other binding limitations provided for under applicable law, the information, documents and data made available on the ECHA website may be reproduced, distributed and/or used, totally or in part, for non-commercial purposes provided that ECHA is acknowledged as the source: "Source: European Chemicals Agency, http://echa.europa.eu/". Such acknowledgement must be included in each copy of the material. ECHA permits and encourages organisations and individuals to create links to the ECHA website under the following cumulative conditions: Links can only be made to webpages that provide a link to the Legal Notice page. | |
Record name | (2-CHLOROPHENYL) (1-HYDROXYCYCLOPENTYL) KETONE | |
Source | FDA Global Substance Registration System (GSRS) | |
URL | https://gsrs.ncats.nih.gov/ginas/app/beta/substances/7428H4566N | |
Description | The FDA Global Substance Registration System (GSRS) enables the efficient and accurate exchange of information on what substances are in regulated products. Instead of relying on names, which vary across regulatory domains, countries, and regions, the GSRS knowledge base makes it possible for substances to be defined by standardized, scientific descriptions. | |
Explanation | Unless otherwise noted, the contents of the FDA website (www.fda.gov), both text and graphics, are not copyrighted. They are in the public domain and may be republished, reprinted and otherwise used freely by anyone without the need to obtain permission from FDA. Credit to the U.S. Food and Drug Administration as the source is appreciated but not required. | |
Disclaimer and Information on In-Vitro Research Products
Please be aware that all articles and product information presented on BenchChem are intended solely for informational purposes. The products available for purchase on BenchChem are specifically designed for in-vitro studies, which are conducted outside of living organisms. In-vitro studies, derived from the Latin term "in glass," involve experiments performed in controlled laboratory settings using cells or tissues. It is important to note that these products are not categorized as medicines or drugs, and they have not received approval from the FDA for the prevention, treatment, or cure of any medical condition, ailment, or disease. We must emphasize that any form of bodily introduction of these products into humans or animals is strictly prohibited by law. It is essential to adhere to these guidelines to ensure compliance with legal and ethical standards in research and experimentation.