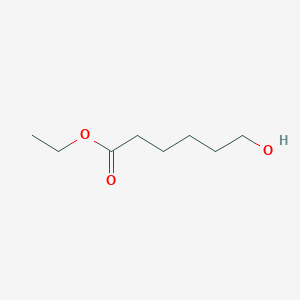
Ethyl 6-hydroxyhexanoate
Overview
Description
Ethyl 6-hydroxyhexanoate is an organic compound with the molecular formula C8H16O3. It is an ethyl ester of 6-hydroxyhexanoic acid and is known for its applications in various chemical syntheses. This compound is often used as a reagent in organic chemistry due to its unique structural properties .
Mechanism of Action
Target of Action
Ethyl 6-hydroxyhexanoate is an organic compound . It is primarily used in the synthesis of model phenol carbonate ester prodrugs . These prodrugs are designed to target specific enzymes in the body that can metabolize them into active drugs .
Mode of Action
It is known that it can be used in the synthesis of alkyl triflates . Alkyl triflates are versatile intermediates in organic synthesis, often used in palladium-catalyzed coupling reactions .
Biochemical Pathways
This compound is involved in the synthesis of model phenol carbonate ester prodrugs It is known that it can be prepared via the hydrolysis of ε-caprolactone .
Pharmacokinetics
It is known that it has a high gastrointestinal absorption and is bbb permeant . Its LogP value indicates moderate lipophilicity, which could influence its distribution in the body .
Result of Action
The result of this compound’s action is the production of model phenol carbonate ester prodrugs . These prodrugs can be metabolized into active drugs in the body
Action Environment
The action of this compound can be influenced by various environmental factors. For instance, its stability can be affected by temperature and pH . Furthermore, its efficacy in the synthesis of other compounds can be influenced by the presence of catalysts and the specific conditions of the reaction .
Biochemical Analysis
Biochemical Properties
Ethyl 6-hydroxyhexanoate plays a role in biochemical reactions. It is used as a reagent in the synthesis of cross-reactive carbohydrate determinants (CCDs) as tools for in vitro allergy diagnosis
Molecular Mechanism
It is known to be used in the synthesis of certain compounds
Preparation Methods
Synthetic Routes and Reaction Conditions: Ethyl 6-hydroxyhexanoate can be synthesized through a two-step process involving the hydrolysis of ε-caprolactone. The first step involves the acid-catalyzed transesterification of ε-caprolactone to form this compound . The reaction conditions typically include the use of an acid catalyst and mild heating to facilitate the transesterification process.
Industrial Production Methods: In industrial settings, the production of this compound follows similar synthetic routes but on a larger scale. The process involves the use of bulk quantities of ε-caprolactone and ethyl alcohol, with the reaction being catalyzed by an acid such as sulfuric acid. The reaction mixture is then subjected to distillation to purify the final product .
Chemical Reactions Analysis
Types of Reactions: Ethyl 6-hydroxyhexanoate undergoes various chemical reactions, including:
Oxidation: This compound can be oxidized to form 6-oxohexanoic acid.
Reduction: It can be reduced to form 6-hydroxyhexanol.
Substitution: this compound can undergo nucleophilic substitution reactions to form various derivatives.
Common Reagents and Conditions:
Oxidation: Common oxidizing agents include potassium permanganate and chromium trioxide.
Reduction: Reducing agents such as lithium aluminum hydride are used.
Substitution: Nucleophiles like sodium ethoxide can be employed for substitution reactions.
Major Products Formed:
Oxidation: 6-oxohexanoic acid.
Reduction: 6-hydroxyhexanol.
Substitution: Various ethyl-substituted hexanoates.
Scientific Research Applications
Ethyl 6-hydroxyhexanoate has several applications in scientific research:
Chemistry: It is used in the synthesis of model phenol carbonate ester prodrugs with fatty acid-like structures.
Medicine: It serves as a precursor in the synthesis of pharmaceutical intermediates.
Industry: this compound is employed in the production of alkyl triflates and other industrial chemicals.
Comparison with Similar Compounds
- Ethyl 6-isocyanatohexanoate
- Methyl 4,4-dimethoxy-3-oxovalerate
- Methyl 6-bromo-2-naphthoate
Comparison: Ethyl 6-hydroxyhexanoate is unique due to its hydroxyl group, which imparts distinct reactivity compared to similar compounds. For instance, ethyl 6-isocyanatohexanoate lacks the hydroxyl group, making it less reactive in nucleophilic substitution reactions. Methyl 4,4-dimethoxy-3-oxovalerate and methyl 6-bromo-2-naphthoate have different functional groups, leading to varied chemical behaviors .
Biological Activity
Ethyl 6-hydroxyhexanoate (CAS No. 5299-60-5) is a compound that has garnered attention in various fields, particularly in biochemistry and polymer chemistry. This article provides a comprehensive overview of its biological activity, including synthesis, enzymatic applications, and potential health implications.
This compound has the molecular formula and a molecular weight of 160.21 g/mol. It is characterized by the following properties:
- Boiling Point : Not available
- Solubility : Very soluble in water (14.0 mg/ml)
- Log P (octanol-water partition coefficient) : 1.09 to 2.33, indicating moderate lipophilicity
- Bioavailability Score : 0.55, suggesting good absorption potential .
Synthesis and Applications
This compound can be synthesized through various methods, including enzymatic processes. One notable study utilized Candida antarctica lipase B for the co-polymerization of this compound with trans-2,5-dihydroxy-3-pentenoic acid methyl ester, resulting in new polyester materials with functional groups that can be further modified . This enzymatic approach highlights the compound's utility in producing biocompatible materials.
Enzymatic Reactions
Research indicates that this compound can serve as a substrate for various enzymes, particularly lipases. Its structure allows it to participate in transesterification reactions, making it valuable in biocatalysis for polymer synthesis. The compound's hydroxyl group enhances its reactivity and compatibility with different catalytic systems .
Toxicological Studies
In terms of safety, this compound has not been classified as harmful by ingestion based on current studies. There is a lack of corroborating evidence from animal or human studies indicating significant toxicity at common exposure levels . This suggests that while it is advisable to handle it with care due to its chemical nature, it does not pose severe health risks under normal conditions.
Case Studies
- Polymer Synthesis : A study demonstrated the successful use of this compound in creating new polyesters through enzymatic polymerization. The resulting materials exhibited functional properties suitable for biomedical applications .
- Biocompatibility Assessments : Another case highlighted its potential as a biocompatible compound when used in polymer formulations aimed at drug delivery systems. The incorporation of this compound into polymer backbones showed promising results in terms of stability and release profiles .
Summary of Research Findings
Properties
IUPAC Name |
ethyl 6-hydroxyhexanoate | |
---|---|---|
Source | PubChem | |
URL | https://pubchem.ncbi.nlm.nih.gov | |
Description | Data deposited in or computed by PubChem | |
InChI |
InChI=1S/C8H16O3/c1-2-11-8(10)6-4-3-5-7-9/h9H,2-7H2,1H3 | |
Source | PubChem | |
URL | https://pubchem.ncbi.nlm.nih.gov | |
Description | Data deposited in or computed by PubChem | |
InChI Key |
HYXRUZUPCFVWAH-UHFFFAOYSA-N | |
Source | PubChem | |
URL | https://pubchem.ncbi.nlm.nih.gov | |
Description | Data deposited in or computed by PubChem | |
Canonical SMILES |
CCOC(=O)CCCCCO | |
Source | PubChem | |
URL | https://pubchem.ncbi.nlm.nih.gov | |
Description | Data deposited in or computed by PubChem | |
Molecular Formula |
C8H16O3 | |
Source | PubChem | |
URL | https://pubchem.ncbi.nlm.nih.gov | |
Description | Data deposited in or computed by PubChem | |
Related CAS |
606130-29-4 | |
Record name | Poly[oxy(1-oxo-1,6-hexanediyl)], α-ethyl-ω-hydroxy- | |
Source | CAS Common Chemistry | |
URL | https://commonchemistry.cas.org/detail?cas_rn=606130-29-4 | |
Description | CAS Common Chemistry is an open community resource for accessing chemical information. Nearly 500,000 chemical substances from CAS REGISTRY cover areas of community interest, including common and frequently regulated chemicals, and those relevant to high school and undergraduate chemistry classes. This chemical information, curated by our expert scientists, is provided in alignment with our mission as a division of the American Chemical Society. | |
Explanation | The data from CAS Common Chemistry is provided under a CC-BY-NC 4.0 license, unless otherwise stated. | |
DSSTOX Substance ID |
DTXSID60326814 | |
Record name | Ethyl 6-hydroxyhexanoate | |
Source | EPA DSSTox | |
URL | https://comptox.epa.gov/dashboard/DTXSID60326814 | |
Description | DSSTox provides a high quality public chemistry resource for supporting improved predictive toxicology. | |
Molecular Weight |
160.21 g/mol | |
Source | PubChem | |
URL | https://pubchem.ncbi.nlm.nih.gov | |
Description | Data deposited in or computed by PubChem | |
CAS No. |
5299-60-5 | |
Record name | Ethyl 6-hydroxyhexanoate | |
Source | CAS Common Chemistry | |
URL | https://commonchemistry.cas.org/detail?cas_rn=5299-60-5 | |
Description | CAS Common Chemistry is an open community resource for accessing chemical information. Nearly 500,000 chemical substances from CAS REGISTRY cover areas of community interest, including common and frequently regulated chemicals, and those relevant to high school and undergraduate chemistry classes. This chemical information, curated by our expert scientists, is provided in alignment with our mission as a division of the American Chemical Society. | |
Explanation | The data from CAS Common Chemistry is provided under a CC-BY-NC 4.0 license, unless otherwise stated. | |
Record name | Ethyl 6-hydroxyhexanoate | |
Source | EPA DSSTox | |
URL | https://comptox.epa.gov/dashboard/DTXSID60326814 | |
Description | DSSTox provides a high quality public chemistry resource for supporting improved predictive toxicology. | |
Retrosynthesis Analysis
AI-Powered Synthesis Planning: Our tool employs the Template_relevance Pistachio, Template_relevance Bkms_metabolic, Template_relevance Pistachio_ringbreaker, Template_relevance Reaxys, Template_relevance Reaxys_biocatalysis model, leveraging a vast database of chemical reactions to predict feasible synthetic routes.
One-Step Synthesis Focus: Specifically designed for one-step synthesis, it provides concise and direct routes for your target compounds, streamlining the synthesis process.
Accurate Predictions: Utilizing the extensive PISTACHIO, BKMS_METABOLIC, PISTACHIO_RINGBREAKER, REAXYS, REAXYS_BIOCATALYSIS database, our tool offers high-accuracy predictions, reflecting the latest in chemical research and data.
Strategy Settings
Precursor scoring | Relevance Heuristic |
---|---|
Min. plausibility | 0.01 |
Model | Template_relevance |
Template Set | Pistachio/Bkms_metabolic/Pistachio_ringbreaker/Reaxys/Reaxys_biocatalysis |
Top-N result to add to graph | 6 |
Feasible Synthetic Routes
Q1: What are the applications of ethyl 6-hydroxyhexanoate in material science?
A1: this compound is a valuable building block in synthesizing polyesters. Research shows that it can be copolymerized with novel monomers like trans-2,5-dihydroxy-3-pentenoic acid methyl ester (DPM) using enzymatic polymerization techniques []. This process yields functional polyesters with molecular weights reaching up to 12,000 g/mol []. These polyesters hold potential for various applications due to their functional groups, allowing for further modifications like grafting [].
Q2: Can this compound be used to create fragrant compounds?
A2: Yes, this compound serves as a precursor to commercially valuable fragrance compounds. One example is its use in the synthesis of ethyl 6-acetoxyhexanoate, also known as Berryflor []. This compound possesses a pleasant raspberry aroma with hints of jasmine and anise, making it suitable for fragrances and flavorings [].
Q3: Are there any alternative synthetic routes for producing this compound?
A3: While commercial sources exist, this compound can be synthesized from readily available starting materials. A common method involves the acid-catalyzed transesterification of ε-caprolactone []. This approach offers a cost-effective alternative to direct purchase, particularly for laboratory-scale syntheses.
Disclaimer and Information on In-Vitro Research Products
Please be aware that all articles and product information presented on BenchChem are intended solely for informational purposes. The products available for purchase on BenchChem are specifically designed for in-vitro studies, which are conducted outside of living organisms. In-vitro studies, derived from the Latin term "in glass," involve experiments performed in controlled laboratory settings using cells or tissues. It is important to note that these products are not categorized as medicines or drugs, and they have not received approval from the FDA for the prevention, treatment, or cure of any medical condition, ailment, or disease. We must emphasize that any form of bodily introduction of these products into humans or animals is strictly prohibited by law. It is essential to adhere to these guidelines to ensure compliance with legal and ethical standards in research and experimentation.