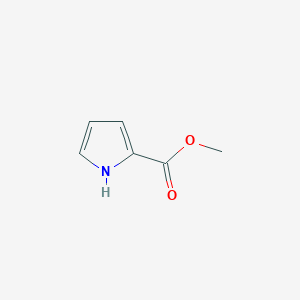
Methyl 1H-pyrrole-2-carboxylate
Overview
Description
Methyl 1H-pyrrole-2-carboxylate is an organic compound with the molecular formula C6H7NO2. It is a derivative of pyrrole, a five-membered aromatic heterocycle containing one nitrogen atom. This compound is known for its applications in organic synthesis and as an intermediate in the production of various pharmaceuticals and agrochemicals .
Mechanism of Action
Target of Action
Methyl 1H-pyrrole-2-carboxylate is a biochemical reagent . .
Pharmacokinetics
It is known that the compound is soluble in water , which may influence its bioavailability.
Action Environment
The action of this compound may be influenced by various environmental factors. For example, it is known to be stable under recommended storage conditions . It is incompatible with strong oxidizing agents, strong acids, and strong bases . These factors may influence the compound’s action, efficacy, and stability.
Biochemical Analysis
Biochemical Properties
It is known to be soluble in various organic solvents such as ethanol, ether, and butanol, but insoluble in water . This solubility profile suggests that it may interact with lipophilic biomolecules within the cell.
Cellular Effects
It is known to cause skin and eye irritation, and may cause respiratory irritation . This suggests that it may interact with cellular membranes and potentially disrupt normal cellular function.
Molecular Mechanism
Its structure suggests that it may interact with biomolecules through hydrogen bonding or other non-covalent interactions .
Temporal Effects in Laboratory Settings
It is known to be stable under recommended storage conditions .
Dosage Effects in Animal Models
It is known to cause skin and eye irritation, suggesting that high doses may cause adverse effects .
Metabolic Pathways
Its structure suggests that it may be metabolized by enzymes that process similar compounds .
Transport and Distribution
Its solubility profile suggests that it may be transported through the cell membrane and distributed within lipophilic compartments of the cell .
Subcellular Localization
Its solubility profile suggests that it may localize within lipophilic compartments of the cell .
Preparation Methods
Synthetic Routes and Reaction Conditions: Methyl 1H-pyrrole-2-carboxylate can be synthesized through several methods. One common method involves the reaction of pyrrole with dimethyl carbonate in the presence of a base. This reaction typically occurs under mild conditions and yields the desired ester product .
Industrial Production Methods: In an industrial setting, the production of this compound often involves the esterification of pyrrole-2-carboxylic acid with methanol in the presence of an acid catalyst. This method is efficient and scalable, making it suitable for large-scale production .
Chemical Reactions Analysis
Types of Reactions: Methyl 1H-pyrrole-2-carboxylate undergoes various chemical reactions, including:
Oxidation: The compound can be oxidized to form pyrrole-2-carboxylic acid.
Reduction: Reduction reactions can convert it into pyrrole-2-methanol.
Substitution: Electrophilic substitution reactions can introduce various substituents onto the pyrrole ring.
Common Reagents and Conditions:
Oxidation: Common oxidizing agents include potassium permanganate and chromium trioxide.
Reduction: Reducing agents such as lithium aluminum hydride or sodium borohydride are often used.
Substitution: Electrophilic reagents like halogens or nitro compounds can be used under acidic or basic conditions.
Major Products:
Oxidation: Pyrrole-2-carboxylic acid.
Reduction: Pyrrole-2-methanol.
Substitution: Various substituted pyrrole derivatives depending on the electrophile used.
Scientific Research Applications
Methyl 1H-pyrrole-2-carboxylate has a wide range of applications in scientific research:
Chemistry: It is used as a building block in the synthesis of more complex organic molecules.
Biology: The compound is used in the study of biological pathways involving pyrrole derivatives.
Medicine: It serves as an intermediate in the synthesis of pharmaceuticals with potential therapeutic effects.
Industry: The compound is used in the production of agrochemicals and dyes
Comparison with Similar Compounds
Methyl 1H-pyrrole-2-carboxylate can be compared with other similar compounds such as:
Pyrrole-2-carboxylic acid: Similar structure but lacks the ester group.
Methyl 1-methylpyrrole-2-carboxylate: Contains an additional methyl group on the nitrogen atom.
Indole derivatives: Share the pyrrole ring but have an additional fused benzene ring
Uniqueness: this compound is unique due to its ester functionality, which makes it more reactive in certain chemical reactions compared to its non-esterified counterparts. This reactivity is particularly useful in organic synthesis and pharmaceutical applications .
Biological Activity
Methyl 1H-pyrrole-2-carboxylate (MPC) is a compound that has garnered interest in the field of medicinal chemistry due to its diverse biological activities. This article delves into the biological activity of MPC, highlighting its synthesis, pharmacological properties, and potential therapeutic applications based on recent research findings.
Chemical Structure and Synthesis
MPC is characterized by its pyrrole ring structure with a carboxylate functional group. The synthesis of MPC typically involves the reaction of pyrrole derivatives with carboxylic acids or their derivatives. The compound can be synthesized through various methods, including:
- Condensation reactions involving pyrrole and methyl chloroacetate.
- Direct esterification of pyrrole-2-carboxylic acid with methanol.
These synthesis routes yield MPC in moderate to high purity, allowing for further biological evaluations.
Biological Activities
MPC exhibits a range of biological activities, which can be categorized as follows:
Antimicrobial Activity
Recent studies have demonstrated that MPC possesses significant antimicrobial properties. It has been shown to inhibit the growth of various bacterial strains, particularly those resistant to conventional antibiotics. In a study assessing the Minimum Inhibitory Concentration (MIC) against Mycobacterium tuberculosis, MPC displayed potent activity with an MIC value below 0.016 μg/mL, indicating its potential as an anti-tuberculosis agent .
Antiviral Properties
MPC has also been investigated for its antiviral activity. It serves as an intermediate in the synthesis of pyrrolyl aryl sulfones, which have been reported to exhibit activity against HIV-1. This suggests that MPC could play a role in developing antiviral therapeutics .
Cytotoxicity and Selectivity
The cytotoxic effects of MPC have been evaluated using various cell lines. Notably, compounds derived from MPC showed low cytotoxicity with IC50 values greater than 64 μg/mL, indicating a favorable selectivity index for therapeutic applications . This low toxicity profile is crucial for the development of safe pharmaceuticals.
Structure-Activity Relationship (SAR)
Understanding the SAR of MPC is essential for optimizing its biological activity. Research indicates that modifications to the pyrrole ring and carboxylate group can significantly influence its potency. For instance, substituents on the pyrrole ring can enhance or diminish antimicrobial efficacy depending on their electronic properties .
Case Studies
Several case studies illustrate the potential applications of MPC:
- Anti-Tuberculosis Activity : A study focused on synthesizing pyrrole-2-carboxamide derivatives revealed that modifications to the carboxamide group significantly improved anti-TB activity, suggesting that similar modifications to MPC could enhance its therapeutic potential against tuberculosis .
- Antiviral Research : In a project aimed at developing new antiviral agents, MPC was utilized as a precursor in synthesizing compounds that exhibited promising activity against HIV-1, demonstrating its utility in antiviral drug development .
- Natural Product Derivatives : Research into naturally occurring pyrrole derivatives has shown that compounds similar to MPC possess various physiological activities, including antioxidant and anti-inflammatory effects, further supporting the exploration of MPC's derivatives for therapeutic use .
Properties
IUPAC Name |
methyl 1H-pyrrole-2-carboxylate | |
---|---|---|
Source | PubChem | |
URL | https://pubchem.ncbi.nlm.nih.gov | |
Description | Data deposited in or computed by PubChem | |
InChI |
InChI=1S/C6H7NO2/c1-9-6(8)5-3-2-4-7-5/h2-4,7H,1H3 | |
Source | PubChem | |
URL | https://pubchem.ncbi.nlm.nih.gov | |
Description | Data deposited in or computed by PubChem | |
InChI Key |
VONGYFFEWFJHNP-UHFFFAOYSA-N | |
Source | PubChem | |
URL | https://pubchem.ncbi.nlm.nih.gov | |
Description | Data deposited in or computed by PubChem | |
Canonical SMILES |
COC(=O)C1=CC=CN1 | |
Source | PubChem | |
URL | https://pubchem.ncbi.nlm.nih.gov | |
Description | Data deposited in or computed by PubChem | |
Molecular Formula |
C6H7NO2 | |
Source | PubChem | |
URL | https://pubchem.ncbi.nlm.nih.gov | |
Description | Data deposited in or computed by PubChem | |
DSSTOX Substance ID |
DTXSID50152407 | |
Record name | Methyl pyrrole-2-carboxylate | |
Source | EPA DSSTox | |
URL | https://comptox.epa.gov/dashboard/DTXSID50152407 | |
Description | DSSTox provides a high quality public chemistry resource for supporting improved predictive toxicology. | |
Molecular Weight |
125.13 g/mol | |
Source | PubChem | |
URL | https://pubchem.ncbi.nlm.nih.gov | |
Description | Data deposited in or computed by PubChem | |
CAS No. |
1193-62-0 | |
Record name | Methyl pyrrole-2-carboxylate | |
Source | ChemIDplus | |
URL | https://pubchem.ncbi.nlm.nih.gov/substance/?source=chemidplus&sourceid=0001193620 | |
Description | ChemIDplus is a free, web search system that provides access to the structure and nomenclature authority files used for the identification of chemical substances cited in National Library of Medicine (NLM) databases, including the TOXNET system. | |
Record name | 1193-62-0 | |
Source | DTP/NCI | |
URL | https://dtp.cancer.gov/dtpstandard/servlet/dwindex?searchtype=NSC&outputformat=html&searchlist=48131 | |
Description | The NCI Development Therapeutics Program (DTP) provides services and resources to the academic and private-sector research communities worldwide to facilitate the discovery and development of new cancer therapeutic agents. | |
Explanation | Unless otherwise indicated, all text within NCI products is free of copyright and may be reused without our permission. Credit the National Cancer Institute as the source. | |
Record name | Methyl pyrrole-2-carboxylate | |
Source | EPA DSSTox | |
URL | https://comptox.epa.gov/dashboard/DTXSID50152407 | |
Description | DSSTox provides a high quality public chemistry resource for supporting improved predictive toxicology. | |
Record name | METHYL 1H-PYRROLE-2-CARBOXYLATE | |
Source | European Chemicals Agency (ECHA) | |
URL | https://echa.europa.eu/information-on-chemicals | |
Description | The European Chemicals Agency (ECHA) is an agency of the European Union which is the driving force among regulatory authorities in implementing the EU's groundbreaking chemicals legislation for the benefit of human health and the environment as well as for innovation and competitiveness. | |
Explanation | Use of the information, documents and data from the ECHA website is subject to the terms and conditions of this Legal Notice, and subject to other binding limitations provided for under applicable law, the information, documents and data made available on the ECHA website may be reproduced, distributed and/or used, totally or in part, for non-commercial purposes provided that ECHA is acknowledged as the source: "Source: European Chemicals Agency, http://echa.europa.eu/". Such acknowledgement must be included in each copy of the material. ECHA permits and encourages organisations and individuals to create links to the ECHA website under the following cumulative conditions: Links can only be made to webpages that provide a link to the Legal Notice page. | |
Record name | METHYL PYRROLE-2-CARBOXYLATE | |
Source | FDA Global Substance Registration System (GSRS) | |
URL | https://gsrs.ncats.nih.gov/ginas/app/beta/substances/3BEG2CK3LU | |
Description | The FDA Global Substance Registration System (GSRS) enables the efficient and accurate exchange of information on what substances are in regulated products. Instead of relying on names, which vary across regulatory domains, countries, and regions, the GSRS knowledge base makes it possible for substances to be defined by standardized, scientific descriptions. | |
Explanation | Unless otherwise noted, the contents of the FDA website (www.fda.gov), both text and graphics, are not copyrighted. They are in the public domain and may be republished, reprinted and otherwise used freely by anyone without the need to obtain permission from FDA. Credit to the U.S. Food and Drug Administration as the source is appreciated but not required. | |
Synthesis routes and methods I
Procedure details
Synthesis routes and methods II
Procedure details
Synthesis routes and methods III
Procedure details
Synthesis routes and methods IV
Procedure details
Synthesis routes and methods V
Procedure details
Retrosynthesis Analysis
AI-Powered Synthesis Planning: Our tool employs the Template_relevance Pistachio, Template_relevance Bkms_metabolic, Template_relevance Pistachio_ringbreaker, Template_relevance Reaxys, Template_relevance Reaxys_biocatalysis model, leveraging a vast database of chemical reactions to predict feasible synthetic routes.
One-Step Synthesis Focus: Specifically designed for one-step synthesis, it provides concise and direct routes for your target compounds, streamlining the synthesis process.
Accurate Predictions: Utilizing the extensive PISTACHIO, BKMS_METABOLIC, PISTACHIO_RINGBREAKER, REAXYS, REAXYS_BIOCATALYSIS database, our tool offers high-accuracy predictions, reflecting the latest in chemical research and data.
Strategy Settings
Precursor scoring | Relevance Heuristic |
---|---|
Min. plausibility | 0.01 |
Model | Template_relevance |
Template Set | Pistachio/Bkms_metabolic/Pistachio_ringbreaker/Reaxys/Reaxys_biocatalysis |
Top-N result to add to graph | 6 |
Feasible Synthetic Routes
Q1: What is the structural significance of Methyl 1H-pyrrole-2-carboxylate?
A1: this compound is characterized by a planar structure. The pyrrole ring and the methoxycarbonyl group (O/C/O/C) are nearly coplanar, with a dihedral angle of 3.6° between them. [] This planarity can influence its interactions in chemical reactions and biological systems.
Q2: How does this compound interact with other molecules in its crystal structure?
A2: In its crystal structure, this compound molecules interact through N-H⋯O hydrogen bonds. The nitrogen atom of the pyrrole ring acts as a hydrogen bond donor to the carbonyl oxygen atom (C=O) of a neighboring molecule. [] This interaction leads to the formation of helical chains along the crystallographic b-axis.
Q3: Can you describe a specific synthetic route where this compound serves as a key starting material?
A3: this compound is a crucial precursor in the synthesis of 2,3-Dihydro-7-methyl-1H-pyrrolizin-1-one, also known as the sex pheromone Danaidone. [] This synthesis involves a Dieckmann reaction on the N-propanoate derivative of this compound.
Q4: Are there any studies investigating the hydrogen bonding capabilities of this compound in comparison to its derivatives?
A5: Yes, studies have delved into the hydrogen bonding patterns of this compound and compared it to derivatives like 2,2-dichloro-1-(1-methyl-1H-pyrrol-2-yl)ethan-1-one and 2-chloro-1-(1H-pyrrol-2-yl)ethan-1-one. [] These studies revealed that while this compound forms chain C(5) motifs through N-H⋯O hydrogen bonds, its derivatives exhibit different motifs, like R12(5) and R21(7), impacting their crystal packing and potentially influencing their physical properties.
Q5: Have computational chemistry methods been applied to this compound or its derivatives?
A6: Indeed, computational methods like ωB97XD/6-311++G(d,p) level of theory have been employed to estimate the interaction energies of hydrogen bonding systems in this compound and its derivatives. [] Such calculations provide insights into the relative stability of different hydrogen bonding arrangements and their potential influence on the compound's properties.
Disclaimer and Information on In-Vitro Research Products
Please be aware that all articles and product information presented on BenchChem are intended solely for informational purposes. The products available for purchase on BenchChem are specifically designed for in-vitro studies, which are conducted outside of living organisms. In-vitro studies, derived from the Latin term "in glass," involve experiments performed in controlled laboratory settings using cells or tissues. It is important to note that these products are not categorized as medicines or drugs, and they have not received approval from the FDA for the prevention, treatment, or cure of any medical condition, ailment, or disease. We must emphasize that any form of bodily introduction of these products into humans or animals is strictly prohibited by law. It is essential to adhere to these guidelines to ensure compliance with legal and ethical standards in research and experimentation.