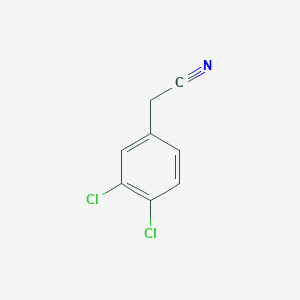
3,4-Dichlorophenylacetonitrile
Overview
Description
3,4-Dichlorophenylacetonitrile is an organic compound with the molecular formula C8H5Cl2N. It is characterized by the presence of two chlorine atoms attached to a phenyl ring and an acetonitrile group. This compound is a white crystalline solid and is known for its applications in various chemical processes .
Mechanism of Action
Target of Action
3,4-Dichlorophenylacetonitrile is primarily used in the synthesis of potent, competitive, and selective non-peptide antagonists of the neurokinin A (NK2) receptor . The NK2 receptor is a member of the tachykinin family of G protein-coupled receptors, which are involved in various physiological processes, including smooth muscle contraction, inflammation, and pain transmission .
Mode of Action
It is known to be a key intermediate in the synthesis of nk2 receptor antagonists . These antagonists work by binding to the NK2 receptor, preventing the natural ligand, neurokinin A, from activating the receptor .
Biochemical Pathways
The biochemical pathways affected by this compound are primarily those involving the NK2 receptor. By inhibiting the activation of this receptor, the compound can potentially affect a variety of physiological processes, including neurotransmission, inflammation, and smooth muscle contraction .
Pharmacokinetics
It is known that the compound has high gastrointestinal absorption and is bbb permeant . The compound is also known to inhibit CYP1A2, an enzyme involved in drug metabolism .
Result of Action
The primary result of the action of this compound is the inhibition of the NK2 receptor. This can lead to a decrease in the physiological effects mediated by this receptor, such as smooth muscle contraction, inflammation, and pain transmission .
Action Environment
The action of this compound can be influenced by various environmental factors. For example, the presence of other drugs that inhibit or induce CYP1A2 could potentially affect the metabolism of the compound . Additionally, conditions that alter gastrointestinal absorption or the function of the blood-brain barrier could also influence the compound’s bioavailability and effects .
Preparation Methods
Synthetic Routes and Reaction Conditions: 3,4-Dichlorophenylacetonitrile can be synthesized from 3,4-dichlorotoluene through a series of chemical reactions. One common method involves the reaction of 3,4-dichlorotoluene with cerium (III) ammonium nitrate and potassium bromide in acetic acid at 80-90°C for 1.5 hours, yielding a 57% product . Another method involves heating 3,4-dichlorotoluene in ethanol and water for 4 hours, resulting in a 79% yield .
Industrial Production Methods: Industrial production of this compound typically involves large-scale synthesis using optimized reaction conditions to maximize yield and purity. The specific details of industrial methods are often proprietary and may vary between manufacturers.
Chemical Reactions Analysis
Types of Reactions: 3,4-Dichlorophenylacetonitrile undergoes various chemical reactions, including:
Oxidation: It can be oxidized to form corresponding carboxylic acids.
Reduction: Reduction reactions can convert it into amines or other reduced forms.
Substitution: It can participate in nucleophilic substitution reactions, where the chlorine atoms are replaced by other nucleophiles.
Common Reagents and Conditions:
Oxidation: Common oxidizing agents include potassium permanganate and chromium trioxide.
Reduction: Lithium aluminum hydride (LiAlH4) is often used for reduction reactions.
Substitution: Nucleophiles such as sodium methoxide or potassium tert-butoxide are commonly used.
Major Products Formed:
Oxidation: Dichlorobenzoic acids.
Reduction: Dichlorophenethylamines.
Substitution: Various substituted phenylacetonitriles depending on the nucleophile used.
Scientific Research Applications
3,4-Dichlorophenylacetonitrile has several applications in scientific research:
Chemistry: It is used as an intermediate in the synthesis of various organic compounds, including pharmaceuticals and agrochemicals.
Medicine: It is used in the development of drugs targeting specific receptors and pathways.
Industry: It serves as a building block for the production of specialty chemicals and materials.
Comparison with Similar Compounds
- 3,4-Dichlorobenzyl cyanide
- 3,4-Dichlorophenethylamine
- 3,4-Dichlorobenzyl alcohol
Comparison: 3,4-Dichlorophenylacetonitrile is unique due to its specific functional groups, which confer distinct reactivity and applications. Compared to 3,4-Dichlorobenzyl cyanide, it has a nitrile group that allows for different chemical transformations. 3,4-Dichlorophenethylamine, on the other hand, is a reduced form and has different biological activities. 3,4-Dichlorobenzyl alcohol is an alcohol derivative with different reactivity and uses .
Properties
IUPAC Name |
2-(3,4-dichlorophenyl)acetonitrile | |
---|---|---|
Source | PubChem | |
URL | https://pubchem.ncbi.nlm.nih.gov | |
Description | Data deposited in or computed by PubChem | |
InChI |
InChI=1S/C8H5Cl2N/c9-7-2-1-6(3-4-11)5-8(7)10/h1-2,5H,3H2 | |
Source | PubChem | |
URL | https://pubchem.ncbi.nlm.nih.gov | |
Description | Data deposited in or computed by PubChem | |
InChI Key |
QWZNCAFWRZZJMA-UHFFFAOYSA-N | |
Source | PubChem | |
URL | https://pubchem.ncbi.nlm.nih.gov | |
Description | Data deposited in or computed by PubChem | |
Canonical SMILES |
C1=CC(=C(C=C1CC#N)Cl)Cl | |
Source | PubChem | |
URL | https://pubchem.ncbi.nlm.nih.gov | |
Description | Data deposited in or computed by PubChem | |
Molecular Formula |
C8H5Cl2N | |
Source | PubChem | |
URL | https://pubchem.ncbi.nlm.nih.gov | |
Description | Data deposited in or computed by PubChem | |
DSSTOX Substance ID |
DTXSID70185959 | |
Record name | (3,4-Dichlorophenyl)acetonitrile | |
Source | EPA DSSTox | |
URL | https://comptox.epa.gov/dashboard/DTXSID70185959 | |
Description | DSSTox provides a high quality public chemistry resource for supporting improved predictive toxicology. | |
Molecular Weight |
186.03 g/mol | |
Source | PubChem | |
URL | https://pubchem.ncbi.nlm.nih.gov | |
Description | Data deposited in or computed by PubChem | |
CAS No. |
3218-49-3 | |
Record name | 3,4-Dichlorobenzeneacetonitrile | |
Source | CAS Common Chemistry | |
URL | https://commonchemistry.cas.org/detail?cas_rn=3218-49-3 | |
Description | CAS Common Chemistry is an open community resource for accessing chemical information. Nearly 500,000 chemical substances from CAS REGISTRY cover areas of community interest, including common and frequently regulated chemicals, and those relevant to high school and undergraduate chemistry classes. This chemical information, curated by our expert scientists, is provided in alignment with our mission as a division of the American Chemical Society. | |
Explanation | The data from CAS Common Chemistry is provided under a CC-BY-NC 4.0 license, unless otherwise stated. | |
Record name | (3,4-Dichlorophenyl)acetonitrile | |
Source | ChemIDplus | |
URL | https://pubchem.ncbi.nlm.nih.gov/substance/?source=chemidplus&sourceid=0003218493 | |
Description | ChemIDplus is a free, web search system that provides access to the structure and nomenclature authority files used for the identification of chemical substances cited in National Library of Medicine (NLM) databases, including the TOXNET system. | |
Record name | 3218-49-3 | |
Source | DTP/NCI | |
URL | https://dtp.cancer.gov/dtpstandard/servlet/dwindex?searchtype=NSC&outputformat=html&searchlist=111725 | |
Description | The NCI Development Therapeutics Program (DTP) provides services and resources to the academic and private-sector research communities worldwide to facilitate the discovery and development of new cancer therapeutic agents. | |
Explanation | Unless otherwise indicated, all text within NCI products is free of copyright and may be reused without our permission. Credit the National Cancer Institute as the source. | |
Record name | (3,4-Dichlorophenyl)acetonitrile | |
Source | EPA DSSTox | |
URL | https://comptox.epa.gov/dashboard/DTXSID70185959 | |
Description | DSSTox provides a high quality public chemistry resource for supporting improved predictive toxicology. | |
Record name | (3,4-dichlorophenyl)acetonitrile | |
Source | European Chemicals Agency (ECHA) | |
URL | https://echa.europa.eu/substance-information/-/substanceinfo/100.019.767 | |
Description | The European Chemicals Agency (ECHA) is an agency of the European Union which is the driving force among regulatory authorities in implementing the EU's groundbreaking chemicals legislation for the benefit of human health and the environment as well as for innovation and competitiveness. | |
Explanation | Use of the information, documents and data from the ECHA website is subject to the terms and conditions of this Legal Notice, and subject to other binding limitations provided for under applicable law, the information, documents and data made available on the ECHA website may be reproduced, distributed and/or used, totally or in part, for non-commercial purposes provided that ECHA is acknowledged as the source: "Source: European Chemicals Agency, http://echa.europa.eu/". Such acknowledgement must be included in each copy of the material. ECHA permits and encourages organisations and individuals to create links to the ECHA website under the following cumulative conditions: Links can only be made to webpages that provide a link to the Legal Notice page. | |
Retrosynthesis Analysis
AI-Powered Synthesis Planning: Our tool employs the Template_relevance Pistachio, Template_relevance Bkms_metabolic, Template_relevance Pistachio_ringbreaker, Template_relevance Reaxys, Template_relevance Reaxys_biocatalysis model, leveraging a vast database of chemical reactions to predict feasible synthetic routes.
One-Step Synthesis Focus: Specifically designed for one-step synthesis, it provides concise and direct routes for your target compounds, streamlining the synthesis process.
Accurate Predictions: Utilizing the extensive PISTACHIO, BKMS_METABOLIC, PISTACHIO_RINGBREAKER, REAXYS, REAXYS_BIOCATALYSIS database, our tool offers high-accuracy predictions, reflecting the latest in chemical research and data.
Strategy Settings
Precursor scoring | Relevance Heuristic |
---|---|
Min. plausibility | 0.01 |
Model | Template_relevance |
Template Set | Pistachio/Bkms_metabolic/Pistachio_ringbreaker/Reaxys/Reaxys_biocatalysis |
Top-N result to add to graph | 6 |
Feasible Synthetic Routes
Q1: What are 3,4-Dichlorophenylacetonitrile (3,4-DCPAN) and 2-Chlorophenylacetonitrile (2-CPAN) and why are they of concern?
A1: 3,4-DCPAN and 2-CPAN are emerging nitrogenous aromatic disinfection byproducts (DBPs) []. They have been detected in both chlorinated and chloraminated drinking water, raising concerns about potential human exposure [].
Q2: How stable are these compounds in drinking water?
A2: Research indicates that both 3,4-DCPAN and 2-CPAN exhibit relatively high stability in drinking water []. They show minimal degradation under varying pH conditions (5-9) and chlorine doses (0-4 mg/L) over a 5-day period []. Additionally, chloramination does not appear to degrade these compounds []. This persistence in treated water underscores the importance of understanding their formation and potential health effects.
Q3: Do common water treatment methods effectively remove 3,4-DCPAN and 2-CPAN?
A3: While conventional water treatment processes are effective in removing many contaminants, the study highlights that 3,4-DCPAN and 2-CPAN demonstrate resistance to some common quenching agents used in these processes []. Specifically, while sodium sulfite shows minimal reactivity, other quenching agents can significantly reduce their concentrations over time []. This suggests that further research is needed to optimize treatment strategies for effectively removing these emerging DBPs from drinking water.
Q4: What is the significance of finding 3,4-DCPAN and 2-CPAN in finished drinking water?
A4: The detection of 3,4-DCPAN (up to 320 ng/L) and 2-CPAN (up to 530 ng/L) in finished drinking water samples from multiple treatment plants suggests that current treatment methods may not be sufficient to completely eliminate these compounds []. This finding emphasizes the need for further investigation into their potential health risks and the development of more effective removal strategies during water treatment.
Disclaimer and Information on In-Vitro Research Products
Please be aware that all articles and product information presented on BenchChem are intended solely for informational purposes. The products available for purchase on BenchChem are specifically designed for in-vitro studies, which are conducted outside of living organisms. In-vitro studies, derived from the Latin term "in glass," involve experiments performed in controlled laboratory settings using cells or tissues. It is important to note that these products are not categorized as medicines or drugs, and they have not received approval from the FDA for the prevention, treatment, or cure of any medical condition, ailment, or disease. We must emphasize that any form of bodily introduction of these products into humans or animals is strictly prohibited by law. It is essential to adhere to these guidelines to ensure compliance with legal and ethical standards in research and experimentation.