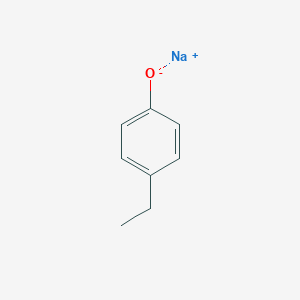
Sodium p-ethylphenolate
- Click on QUICK INQUIRY to receive a quote from our team of experts.
- With the quality product at a COMPETITIVE price, you can focus more on your research.
Overview
Description
Sodium p-ethylphenolate, also known as sodium 4-ethylphenolate, is an organic compound with the molecular formula C8H9NaO. It is a derivative of phenol where the hydrogen atom in the hydroxyl group is replaced by a sodium ion. This compound is commonly used in various chemical reactions and industrial applications due to its unique properties.
Preparation Methods
Synthetic Routes and Reaction Conditions
Sodium p-ethylphenolate can be synthesized through several methods. One common method involves the nucleophilic aromatic substitution of aryl halides. In this process, an aryl halide reacts with sodium hydroxide to form the sodium salt of the phenol . Another method involves the ipso-hydroxylation of arylboronic acids using aqueous hydrogen peroxide as the oxidant and H2O2/HBr as the reagent .
Industrial Production Methods
Industrial production of phenol, 4-ethyl-, sodium salt typically involves the reaction of 4-ethylphenol with sodium hydroxide. This reaction is carried out under controlled conditions to ensure high yield and purity of the product .
Chemical Reactions Analysis
Types of Reactions
Sodium p-ethylphenolate undergoes various chemical reactions, including:
Oxidation: It can be oxidized to form quinones.
Reduction: Reduction reactions can convert quinones back to hydroquinones.
Substitution: Electrophilic aromatic substitution reactions are common, where the aromatic ring undergoes substitution reactions with electrophiles.
Common Reagents and Conditions
Oxidation: Common oxidizing agents include hydrogen peroxide and other peroxides.
Reduction: Reducing agents such as sodium borohydride can be used.
Substitution: Electrophilic reagents like bromine and nitric acid are commonly used.
Major Products Formed
Oxidation: Quinones and hydroquinones.
Reduction: Hydroquinones.
Substitution: Various substituted phenols, such as brominated or nitrated phenols.
Scientific Research Applications
Sodium p-ethylphenolate has a wide range of applications in scientific research:
Chemistry: It is used as a reagent in organic synthesis and as a precursor for the synthesis of other compounds.
Biology: It is used in the study of enzyme mechanisms and as a model compound for studying phenolic compounds.
Industry: It is used in the production of polymers, resins, and other industrial chemicals.
Mechanism of Action
The mechanism of action of phenol, 4-ethyl-, sodium salt involves its ability to act as a nucleophile in various chemical reactions. It can donate electrons to electrophiles, facilitating substitution reactions on the aromatic ring. Additionally, it can undergo oxidation and reduction reactions, making it a versatile compound in organic synthesis .
Comparison with Similar Compounds
Sodium p-ethylphenolate can be compared with other similar compounds such as:
Phenol: The parent compound, which lacks the ethyl group.
4-Methylphenol (p-Cresol): Similar structure but with a methyl group instead of an ethyl group.
4-Propylphenol: Similar structure but with a propyl group instead of an ethyl group.
This compound is unique due to the presence of the ethyl group, which can influence its reactivity and physical properties compared to other phenolic compounds .
Properties
CAS No. |
19277-91-9 |
---|---|
Molecular Formula |
C8H9NaO |
Molecular Weight |
144.15 g/mol |
IUPAC Name |
sodium;4-ethylphenolate |
InChI |
InChI=1S/C8H10O.Na/c1-2-7-3-5-8(9)6-4-7;/h3-6,9H,2H2,1H3;/q;+1/p-1 |
InChI Key |
CJAHZFPECKDBKK-UHFFFAOYSA-M |
SMILES |
CCC1=CC=C(C=C1)[O-].[Na+] |
Isomeric SMILES |
CCC1=CC=C(C=C1)[O-].[Na+] |
Canonical SMILES |
CCC1=CC=C(C=C1)[O-].[Na+] |
Key on ui other cas no. |
19277-91-9 |
Related CAS |
123-07-9 (Parent) |
Origin of Product |
United States |
Disclaimer and Information on In-Vitro Research Products
Please be aware that all articles and product information presented on BenchChem are intended solely for informational purposes. The products available for purchase on BenchChem are specifically designed for in-vitro studies, which are conducted outside of living organisms. In-vitro studies, derived from the Latin term "in glass," involve experiments performed in controlled laboratory settings using cells or tissues. It is important to note that these products are not categorized as medicines or drugs, and they have not received approval from the FDA for the prevention, treatment, or cure of any medical condition, ailment, or disease. We must emphasize that any form of bodily introduction of these products into humans or animals is strictly prohibited by law. It is essential to adhere to these guidelines to ensure compliance with legal and ethical standards in research and experimentation.