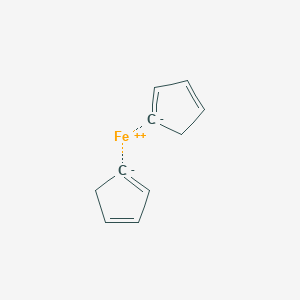
Cyclopenta-1,3-diene;iron(2+)
- Click on QUICK INQUIRY to receive a quote from our team of experts.
- With the quality product at a COMPETITIVE price, you can focus more on your research.
Overview
Description
Cyclopenta-1,3-diene;iron(2+), commonly known as ferrocene (IUPAC: bis(η⁵-cyclopentadienyl)iron), is a prototypical organometallic compound with a sandwich structure comprising an Fe²⁺ ion coordinated between two cyclopentadienyl (Cp) rings . Its molecular formula is $ \text{C}{10}\text{H}{10}\text{Fe} $, and it has a molecular weight of 186.04 g/mol. Ferrocene is notable for its high thermal stability, aromatic character, and redox activity, making it a cornerstone in catalysis, materials science, and medicinal chemistry .
Preparation Methods
Precursor Synthesis: Cyclopentadiene Production
The synthesis of ferrocene necessitates high-purity cyclopentadiene (C₅H₆), a highly reactive diene that dimerizes spontaneously at room temperature. Industrial and laboratory methods for cyclopentadiene production are critical precursors to ferrocene synthesis.
Thermal Cracking of Dicyclopentadiene
Dicyclopentadiene (C₁₀H₁₂), the stable dimer of cyclopentadiene, undergoes retro-Diels-Alder decomposition at elevated temperatures. Industrial-scale production involves steam cracking of naphtha or coal tar, yielding dicyclopentadiene as a byproduct . Laboratory methods employ fractional distillation at 180°C under inert atmospheres to isolate monomeric cyclopentadiene, which is collected at 40–42°C and stored at –20°C to retard redimerization .
Key Parameters for Thermal Cracking
Parameter | Industrial Process | Laboratory Process |
---|---|---|
Temperature | 180–200°C | 180°C (Vigreux column) |
Yield | ~14 kg/ton naphtha | 70–80% monomer recovery |
Purity | 95–98% | ≥99% (after distillation) |
Traditional Laboratory Synthesis
The classical synthesis of ferrocene involves the reaction of cyclopentadienyl anions with iron(II) salts under rigorously anhydrous conditions. Two principal methodologies dominate academic settings.
Alkali Metal-Mediated Synthesis
Developed by Kealy and Pauson in 1951, this method employs sodium cyclopentadienide (NaC₅H₅) and iron(II) chloride in tetrahydrofuran (THF) or dimethoxyethane (DME) .
Procedure
-
Deprotonation : Cyclopentadiene is treated with sodium hydride (NaH) or potassium hydroxide (KOH) in DME to form the cyclopentadienyl anion:
C5H6+KOH→C5H5−K++H2O . -
Coordination : Iron(II) chloride (FeCl₂) is added dropwise, facilitating ligand substitution:
2C5H5−+FeCl2→Fe(C5H5)2+2Cl− . -
Workup : The reaction mixture is quenched with hydrochloric acid, and crude ferrocene is isolated via filtration. Purification by sublimation at 150°C under vacuum yields orange crystals (85–90% purity) .
Optimization Insights
-
Solvent Choice : DME enhances anion stability compared to THF, reducing side reactions .
-
Iron Source : Anhydrous FeCl₂ is critical; hydrated salts (e.g., FeCl₂·4H₂O) necessitate pre-drying to avoid hydrolysis .
Grignard Reagent Alternative
Ferrocene can also be synthesized using cyclopentadienyl magnesium bromide (CpMgBr) and iron(III) chloride, though this route is less common due to lower yields (60–70%) .
Industrial-Scale Production
Commercial ferrocene production prioritizes cost efficiency and scalability, employing distinct reagents and continuous-flow systems.
Iron(II) Ethoxide Route
In a patented industrial process, iron(II) ethoxide (Fe(OEt)₂) reacts directly with cyclopentadiene in a nonpolar solvent (e.g., toluene) . The exothermic reaction (ΔH ≈ –120 kJ/mol) proceeds at 80–100°C, achieving 90–95% conversion within 2 hours.
Advantages
-
Eliminates alkali metals, reducing waste generation.
-
Continuous distillation removes ethanol byproduct, driving equilibrium toward ferrocene .
Electrochemical Synthesis
Recent advancements utilize electrolytic reduction of iron(III) salts in the presence of cyclopentadiene. A divided cell with a platinum anode and iron cathode achieves 80% Faradaic efficiency at 1.5 V .
Advanced Catalytic Methods
Zeolite-Supported Synthesis
Grafting ferrocene derivatives onto HY zeolites enables site-isolated iron centers for catalytic applications. The reaction of ferrocene with Brønsted acid sites in dehydrated HY zeolite at 423 K yields a mononuclear surface complex, [(≡SiO)₂Fe(C₅H₆)], characterized by XAFS and IR spectroscopy .
Reactivity Profile
-
CO Adsorption : The grafted complex reacts with CO at 323 K, forming a carbonyl adduct (ν(CO) = 1980 cm⁻¹) .
-
Thermal Stability : Decomposition occurs above 500 K, releasing cyclopentadiene and depositing iron oxides .
Purification and Characterization
Sublimation
Laboratory-scale purification exploits ferrocene’s high volatility. Sublimation at 150°C (10⁻² mbar) yields ultrapure crystals (99.9%), as confirmed by melting point (172–174°C) and NMR spectroscopy .
Recrystallization
Alternative purification via recrystallization from hexane or petroleum ether affords lower yields (70–75%) but is preferable for large-scale batches .
Comparative Analysis of Purification Methods
Method | Yield (%) | Purity (%) | Time (h) |
---|---|---|---|
Sublimation | 85–90 | 99.9 | 4–6 |
Recrystallization | 70–75 | 98–99 | 8–12 |
Mechanistic and Kinetic Considerations
The formation of ferrocene proceeds via a two-step nucleophilic substitution mechanism:
-
Ligand Dissociation : FeCl₂ undergoes partial dissociation in polar aprotic solvents, generating [FeCl]⁺ intermediates .
-
Anion Coordination : Cyclopentadienyl anions sequentially displace chloride ligands, forming the sandwich complex .
Kinetic studies reveal a second-order dependence on [C₅H₅⁻], with an activation energy of 45 kJ/mol in DME .
Chemical Reactions Analysis
Cyclopenta-1,3-diene;iron(2+) undergoes various reactions typical of aromatic compounds:
Substitution Reactions: Similar to benzene, ferrocene undergoes electrophilic aromatic substitution reactions.
Reduction: Cyclopenta-1,3-diene;iron(2+) can be reduced back from the ferrocenium cation under appropriate conditions.
Major Products:
Acetylferrocene: Formed through the acetylation of ferrocene.
Ferrocenium Cation: Formed through the oxidation of ferrocene.
Scientific Research Applications
Cyclopenta-1,3-diene;iron(2+) and its derivatives have found applications across various scientific fields:
Chemistry: Used as a catalyst in various organic reactions, including cross-coupling and hydrogenation.
Biology and Medicine: Cyclopenta-1,3-diene;iron(2+) derivatives are being explored for their potential as anticancer, antiviral, and antimicrobial agents.
Electrochemistry: Cyclopenta-1,3-diene;iron(2+)-based compounds are used in the development of electrocatalysts due to their excellent redox properties.
Mechanism of Action
The mechanism by which ferrocene exerts its effects, particularly in biological systems, often involves the production of reactive oxygen species (ROS). The Fenton reaction, which generates hydroxyl radicals, is a key pathway. This mechanism is crucial in the context of ferrocene-based drugs, where the generation of ROS can induce apoptosis in cancer cells .
Comparison with Similar Compounds
Structural and Electronic Properties
Table 1: Key Properties of Ferrocene and Derivatives
Electronic Differences :
- Aromatic Stability: The Cp rings in ferrocene are aromatic (6 π-electrons), contributing to its stability . Derivatives like ethylferrocene retain aromaticity but exhibit altered electron density due to alkyl substituents, which donate electrons and increase solubility in nonpolar solvents .
Diels-Alder Reactivity
- Ferrocene : The Cp rings participate in Diels-Alder reactions as electron-rich dienes. For example, cyclopenta-1,3-diene reacts with anthracene to form complex bicyclic structures .
- Substituted Derivatives : Electron-withdrawing groups (e.g., acrylate in ferrocenylmethyl acrylate) reduce diene reactivity, while electron-donating groups (e.g., ethyl in ethylferrocene) enhance it .
Stability Considerations :
- Ferrocene is air-stable, but derivatives like chloromercuriferrocene require inert storage due to mercury’s sensitivity to light and moisture .
- The pKa of cyclopentadiene (~15) facilitates deprotonation to form the aromatic Cp⁻ ligand, whereas anti-aromatic systems (e.g., cycloheptatrienyl anion) have pKa > 36, making them difficult to isolate .
Biological Activity
Cyclopenta-1,3-diene;iron(2+), commonly known as ferrocene, is an organometallic compound with significant biological activity. Its unique structure, characterized by a central iron atom sandwiched between two cyclopentadienyl anions, contributes to its stability and reactivity. This article delves into the biological activity of this compound, including its mechanisms of action, potential therapeutic applications, and relevant research findings.
Overview of Cyclopenta-1,3-diene;iron(2+)
- Chemical Structure : Ferrocene has the formula Fe(C₅H₅)₂, where the iron atom is coordinated to two cyclopentadienyl rings.
- Synthesis : It is typically synthesized through the reaction of sodium cyclopentadienide with iron(II) chloride or via industrial methods involving iron(II) ethoxide and cyclopentadiene .
The biological activity of cyclopenta-1,3-diene;iron(2+) is primarily attributed to its ability to undergo redox reactions:
- Redox Properties : The iron center can exist in different oxidation states (Fe(0) and Fe(II)), allowing it to participate in electron transfer processes that are crucial for various biochemical reactions.
- Coordination Chemistry : The coordination of cyclopentadienyl ligands stabilizes the iron center and modulates its reactivity, influencing interactions with biomolecules.
Anticancer Activity
Research has indicated that ferrocene derivatives exhibit promising anticancer properties:
- Mechanisms : These compounds can induce apoptosis in cancer cells through oxidative stress mechanisms. The iron center may play a role in mimicking essential metal ions in biological systems, facilitating interactions with cellular targets.
- Case Studies : In vitro studies have shown that ferrocene derivatives can inhibit tumor growth in various cancer cell lines, suggesting their potential as therapeutic agents .
Antimicrobial Properties
Ferrocene and its derivatives have also been studied for their antimicrobial activity:
- Research Findings : Some studies have demonstrated that these compounds can inhibit the growth of certain bacterial strains, possibly through disruption of bacterial cell membranes or interference with metabolic pathways .
Comparative Analysis with Similar Compounds
The biological activity of cyclopenta-1,3-diene;iron(2+) can be compared with other metallocenes such as nickelocene and cobaltocene:
Compound | Biological Activity | Mechanism of Action |
---|---|---|
Cyclopenta-1,3-diene;iron(2+) | Anticancer, antimicrobial | Redox reactions, apoptosis induction |
Nickelocene | Limited data on anticancer effects | Similar redox properties |
Cobaltocene | Potential for catalytic reactions | Electron transfer processes |
Table 1: Summary of Biological Studies on Cyclopenta-1,3-diene;iron(2+)
Future Directions
The ongoing research into cyclopenta-1,3-diene;iron(2+) suggests several avenues for future exploration:
- Therapeutic Development : Further investigation into its potential as an anticancer agent could lead to new treatment options.
- Mechanistic Studies : Understanding the precise biochemical pathways affected by this compound will enhance its application in medicinal chemistry.
- Formulation Studies : Developing effective delivery systems for ferrocene derivatives could improve their bioavailability and therapeutic efficacy.
Q & A
Basic Research Questions
Q. What spectroscopic and crystallographic techniques are recommended for confirming the structural integrity of Cyclopenta-1,3-diene;iron(2+) complexes?
- Methodology : Use X-ray crystallography to determine bond lengths and angles in the solid state. 1H/13C NMR in solution-phase analysis identifies cyclopentadienyl ligands via aromatic proton signals (δ 4.0–4.5 ppm). Infrared spectroscopy confirms functional groups, while Mössbauer spectroscopy characterizes the iron center's oxidation state and coordination environment .
Q. How does the aromatic nature of the cyclopentadienyl ligand influence the stability of Cyclopenta-1,3-diene;iron(2+)?
- Analysis : The 6 π-electron aromatic system stabilizes the complex through delocalized bonding. Stability decreases in polar solvents (e.g., DMSO) due to ligand dissociation. Optimize experiments using non-polar solvents (e.g., dichloromethane) and inert atmospheres to prevent oxidation. Aromaticity also enables predictable substitution in derivative synthesis .
Advanced Research Questions
Q. What experimental design considerations are critical for studying redox-mediated biological effects of Cyclopenta-1,3-diene;iron(2+) in cancer models?
- Protocol :
- Control oxygen levels (hypoxic vs. normoxic).
- Use ferrocene/ferrocenium analogs as controls.
- Monitor ROS with fluorescent probes (e.g., DCFH-DA) and validate with antioxidants (N-acetylcysteine).
- Employ flow cytometry for apoptosis and Western blotting for ferroptosis markers (GPX4, ACSL4).
- Standardize culture media to avoid iron chelation artifacts .
Q. How can density functional theory (DFT) resolve discrepancies in electrochemical properties of Cyclopenta-1,3-diene;iron(2+) derivatives?
- Computational Workflow :
Geometry optimization (B3LYP/LANL2DZ basis set for Fe).
Solvent effects via PCM model.
Redox potential prediction using ΔSCF method.
Frontier orbital analysis (HOMO-LUMO gaps).
Validate against cyclic voltammetry (0.1M TBAPF6, 100 mV/s).
- Address discrepancies by analyzing ligand substitutions, solvent reorganization, and counterion interactions. Reference Colle-Salvetti correlation-energy methods for electron density calculations .
Q. How to detect solvent polarity effects on electrochemical behavior of Cyclopenta-1,3-diene;iron(2+) complexes?
- Approach :
- Cyclic voltammetry across solvent series (dichloromethane to acetonitrile).
- UV-Vis spectroelectrochemistry for redox-induced absorbance changes.
- Kirkwood-Onsager equations for dielectric constant measurements.
- Temperature-dependent studies (298–343 K) to isolate thermal effects.
- Significant ΔE1/2 shifts (>50 mV/solvent unit) indicate polarity dependence .
Comparative Research Questions
Q. What distinguishes Cyclopenta-1,3-diene;iron(2+) from analogous metallocenes (e.g., cobaltocene) in catalytic applications?
- Key Differences : Iron’s lower electronegativity enhances electron-donating capacity, improving catalytic activity in hydrogenation and polymerization. Use X-ray absorption spectroscopy (XAS) to compare metal-ligand charge transfer and cyclic voltammetry to assess redox stability .
Q. Data Analysis & Contradictions
Q. How to reconcile conflicting reports on the solubility of Cyclopenta-1,3-diene;iron(2+) in polar aprotic solvents?
- Resolution : Conduct systematic solubility tests (e.g., gravimetric analysis) under controlled humidity and temperature. Compare with computational solubility parameters (Hansen solubility model) and validate via 1H NMR peak broadening in different solvents .
Q. Methodological Tables
Table 1 : Key Spectroscopic Signatures of Cyclopenta-1,3-diene;iron(2+)
Technique | Characteristic Feature | Application |
---|---|---|
1H NMR | δ 4.0–4.5 ppm (cyclopentadienyl protons) | Ligand confirmation |
Mössbauer | Quadrupole splitting ΔE ≈ 2.3 mm/s (Fe²⁺) | Oxidation state analysis |
IR | ~3100 cm⁻¹ (C–H stretch, aromatic) | Functional group identification |
Table 2 : Experimental Design for Redox Studies in Cancer Cells
Parameter | Consideration | Reference Technique |
---|---|---|
Oxygen Control | Hypoxia chamber vs. ambient O₂ | Fluorescence microscopy |
ROS Detection | DCFH-DA probe, confocal imaging | Flow cytometry |
Ferroptosis Markers | GPX4/ACSL4 Western blot | Quantitative PCR |
Properties
CAS No. |
102-54-5 |
---|---|
Molecular Formula |
C10H2Fe-8 |
Molecular Weight |
177.97 g/mol |
IUPAC Name |
cyclopenta-1,3-diene;iron(2+) |
InChI |
InChI=1S/2C5H.Fe/c2*1-2-4-5-3-1;/h2*1H;/q2*-5;+2 |
InChI Key |
NHMCPAQVYGMWBQ-UHFFFAOYSA-N |
SMILES |
C1C=CC=[C-]1.C1C=CC=[C-]1.[Fe+2] |
Canonical SMILES |
[CH-]1[C-]=[C-][C-]=[C-]1.[CH-]1[C-]=[C-][C-]=[C-]1.[Fe+2] |
boiling_point |
480 °F at 760 mmHg (NTP, 1992) 249 °C 480 °F |
Color/Form |
Orange, crystalline solid Orange needles from methanol or ethanol |
melting_point |
343 to 345 °F (sublimes) (NTP, 1992) 173-174 °C 173 °C 343 °F |
Key on ui other cas no. |
102-54-5 |
physical_description |
Ferrocene appears as orange crystalline solid or orange-yellow powder. Sublimes above 212 °F. Camphor odor. (NTP, 1992) Orange, crystalline solid with a camphor-like odor; [NIOSH] ORANGE CRYSTALS WITH CHARACTERISTIC ODOUR. Orange, crystalline solid or orange-yellow powder with a camphor-like odor. Orange, crystalline solid with a camphor-like odor. |
Pictograms |
Flammable; Irritant; Environmental Hazard |
shelf_life |
Unusually stable |
solubility |
less than 0.1 mg/mL at 70 °F (NTP, 1992) Slightly sol in petroleum ether Dissolves in dilute nitric and concentrated sulfuric acids; sol in alcohol, ether; practically insoluble in ... 10% sodium hydroxide, and concentrated boiling hydrochloric acid. Solubility at 25 °C: 19 g/100 g benzene; 10 g/100 g catalytically cracked gasoline; 9 g/100 g straight run gasoline; 6 g/100 g jet fuel (jp-4); and 5 g/100 g diesel fuel. 20 mg/mL in 2-methoxyethanol; 7 mg/ml in ethanol <0.1 mg/mL in water Solubility in water: none Insoluble |
Synonyms |
Bis(cyclopentadienyl)iron; Bis(η-cyclopentadienyl)iron; Bis(η5-cyclopentadienyl)iron; Catane TM; Di-2,4-cyclopentadien-1-yliron; Di-π-cyclopentadienyl Iron; Dicyclopentadienyliron; Ferrotsen; Iron Bis(cyclopentadienide); Iron Dicyclopentadienyl; bis( |
vapor_pressure |
0.0066 kPa at 40 °C; 0.34 kPa at 100 °C Vapor pressure, Pa at 40 °C: 4 |
Origin of Product |
United States |
Disclaimer and Information on In-Vitro Research Products
Please be aware that all articles and product information presented on BenchChem are intended solely for informational purposes. The products available for purchase on BenchChem are specifically designed for in-vitro studies, which are conducted outside of living organisms. In-vitro studies, derived from the Latin term "in glass," involve experiments performed in controlled laboratory settings using cells or tissues. It is important to note that these products are not categorized as medicines or drugs, and they have not received approval from the FDA for the prevention, treatment, or cure of any medical condition, ailment, or disease. We must emphasize that any form of bodily introduction of these products into humans or animals is strictly prohibited by law. It is essential to adhere to these guidelines to ensure compliance with legal and ethical standards in research and experimentation.