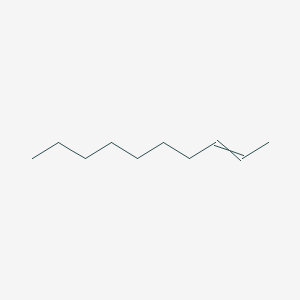
trans-2-Decene
Overview
Description
trans-2-Decene is an unsaturated hydrocarbon with a carbon-carbon double bond located at the second carbon atom in the chain. It is a member of the alkene family and has the molecular formula C10H20. The “trans” designation indicates that the hydrogen atoms attached to the carbon atoms of the double bond are on opposite sides, giving the molecule a more linear structure compared to its “cis” counterpart.
Preparation Methods
Synthetic Routes and Reaction Conditions: trans-2-Decene can be synthesized through various methods, including:
Dehydration of Alcohols: One common method involves the dehydration of 2-decanol using an acid catalyst such as sulfuric acid or phosphoric acid. The reaction is typically carried out at elevated temperatures to facilitate the removal of water and formation of the double bond.
Dehydrohalogenation of Alkyl Halides: Another method involves the elimination of hydrogen halide from 2-decyl halides using a strong base like potassium tert-butoxide in a suitable solvent such as dimethyl sulfoxide.
Industrial Production Methods: Industrial production of this compound often involves the catalytic dehydrogenation of decane. This process uses metal catalysts such as platinum or palladium at high temperatures to remove hydrogen atoms and form the double bond.
Chemical Reactions Analysis
Types of Reactions: trans-2-Decene undergoes various chemical reactions, including:
Oxidation: It can be oxidized to form epoxides or diols using oxidizing agents like peracids or osmium tetroxide.
Hydrogenation: The double bond can be hydrogenated to form decane using hydrogen gas in the presence of a metal catalyst such as palladium on carbon.
Halogenation: this compound reacts with halogens like bromine or chlorine to form vicinal dihalides.
Hydrohalogenation: Addition of hydrogen halides like hydrogen chloride or hydrogen bromide across the double bond results in the formation of alkyl halides.
Common Reagents and Conditions:
Oxidation: Peracids (e.g., m-chloroperbenzoic acid), osmium tetroxide.
Hydrogenation: Hydrogen gas, palladium on carbon catalyst.
Halogenation: Bromine, chlorine.
Hydrohalogenation: Hydrogen chloride, hydrogen bromide.
Major Products:
Oxidation: Epoxides, diols.
Hydrogenation: Decane.
Halogenation: Vicinal dihalides.
Hydrohalogenation: Alkyl halides.
Scientific Research Applications
trans-2-Decene has a wide range of applications in scientific research, including:
Chemistry: It is used as a starting material for the synthesis of various organic compounds and as a reagent in organic reactions.
Biology: this compound is studied for its potential biological activities and interactions with biological molecules.
Medicine: Research is ongoing to explore its potential therapeutic applications and effects on human health.
Industry: It is used in the production of lubricants, surfactants, and other industrial chemicals.
Mechanism of Action
The mechanism of action of trans-2-Decene involves its ability to participate in various chemical reactions due to the presence of the carbon-carbon double bond. This double bond is a site of high electron density, making it reactive towards electrophiles. The molecular targets and pathways involved depend on the specific reaction and conditions used.
Comparison with Similar Compounds
cis-2-Decene: The “cis” isomer has hydrogen atoms on the same side of the double bond, resulting in a more bent structure.
1-Decene: An alkene with the double bond at the first carbon atom.
3-Decene: An alkene with the double bond at the third carbon atom.
Uniqueness: trans-2-Decene is unique due to its linear structure, which affects its physical properties and reactivity compared to its “cis” isomer and other positional isomers. The “trans” configuration results in a higher melting point and different reactivity patterns in chemical reactions.
Biological Activity
trans-2-Decene, a long-chain unsaturated hydrocarbon, has garnered attention in recent years for its potential biological activities. This article explores its biological activity, mechanisms of action, and applications based on diverse research findings.
Chemical Structure and Properties
This compound is an alkene with the molecular formula characterized by a double bond between the second and third carbon atoms in the chain. Its structure allows it to participate in various chemical reactions, making it a valuable compound in organic synthesis and biological studies.
The biological activity of this compound can be attributed to its ability to interact with various biological molecules. The presence of the carbon-carbon double bond provides a site for electrophilic reactions, which can influence cellular processes. Key mechanisms include:
- Interaction with Enzymes : this compound may interact with enzymes involved in lipid metabolism, potentially modulating metabolic pathways and influencing gene expression related to lipid profiles.
- Cell Signaling : The compound has been shown to affect cell signaling pathways by interacting with membrane receptors, which can lead to alterations in cellular responses and behaviors.
Biological Activity
Research indicates that this compound exhibits a range of biological activities:
- Antimicrobial Properties : Studies have shown that compounds containing similar structural features to this compound exhibit potent antibacterial activities. This suggests that this compound may also possess antimicrobial properties, making it a candidate for further investigation in antibiotic development .
- Influence on Lipid Metabolism : The compound has been implicated in metabolic processes that regulate lipid profiles within cells. Its interactions with metabolic enzymes may lead to significant changes in lipid signaling pathways, which are crucial for maintaining cellular homeostasis.
- Ecological Applications : Due to its potential role as a pheromone or signaling molecule in certain species, this compound could have applications in pest management and ecological studies.
Table 1: Summary of Biological Activities of this compound
Case Study: Antibacterial Activity
A study conducted by Theodorakis et al. explored the synthesis of trans-bicyclo[4.4.0]decanes, which include structural analogs of this compound. These compounds demonstrated significant antibacterial activity against resistant strains of bacteria, highlighting the potential of this compound as a scaffold for developing new antibiotics .
Case Study: Lipid Metabolism
Research focusing on the metabolic pathways influenced by this compound revealed its capacity to modulate key enzymes involved in lipid metabolism. This modulation can lead to altered gene expression patterns that affect overall cellular function and health.
Properties
IUPAC Name |
dec-2-ene | |
---|---|---|
Source | PubChem | |
URL | https://pubchem.ncbi.nlm.nih.gov | |
Description | Data deposited in or computed by PubChem | |
InChI |
InChI=1S/C10H20/c1-3-5-7-9-10-8-6-4-2/h3,5H,4,6-10H2,1-2H3 | |
Source | PubChem | |
URL | https://pubchem.ncbi.nlm.nih.gov | |
Description | Data deposited in or computed by PubChem | |
InChI Key |
YKNMBTZOEVIJCM-UHFFFAOYSA-N | |
Source | PubChem | |
URL | https://pubchem.ncbi.nlm.nih.gov | |
Description | Data deposited in or computed by PubChem | |
Canonical SMILES |
CCCCCCCC=CC | |
Source | PubChem | |
URL | https://pubchem.ncbi.nlm.nih.gov | |
Description | Data deposited in or computed by PubChem | |
Molecular Formula |
C10H20 | |
Source | PubChem | |
URL | https://pubchem.ncbi.nlm.nih.gov | |
Description | Data deposited in or computed by PubChem | |
DSSTOX Substance ID |
DTXSID90860337 | |
Record name | 2-Decene | |
Source | EPA DSSTox | |
URL | https://comptox.epa.gov/dashboard/DTXSID90860337 | |
Description | DSSTox provides a high quality public chemistry resource for supporting improved predictive toxicology. | |
Molecular Weight |
140.27 g/mol | |
Source | PubChem | |
URL | https://pubchem.ncbi.nlm.nih.gov | |
Description | Data deposited in or computed by PubChem | |
CAS No. |
6816-17-7 | |
Record name | 2-Decene | |
Source | EPA DSSTox | |
URL | https://comptox.epa.gov/dashboard/DTXSID90860337 | |
Description | DSSTox provides a high quality public chemistry resource for supporting improved predictive toxicology. | |
Synthesis routes and methods
Procedure details
Retrosynthesis Analysis
AI-Powered Synthesis Planning: Our tool employs the Template_relevance Pistachio, Template_relevance Bkms_metabolic, Template_relevance Pistachio_ringbreaker, Template_relevance Reaxys, Template_relevance Reaxys_biocatalysis model, leveraging a vast database of chemical reactions to predict feasible synthetic routes.
One-Step Synthesis Focus: Specifically designed for one-step synthesis, it provides concise and direct routes for your target compounds, streamlining the synthesis process.
Accurate Predictions: Utilizing the extensive PISTACHIO, BKMS_METABOLIC, PISTACHIO_RINGBREAKER, REAXYS, REAXYS_BIOCATALYSIS database, our tool offers high-accuracy predictions, reflecting the latest in chemical research and data.
Strategy Settings
Precursor scoring | Relevance Heuristic |
---|---|
Min. plausibility | 0.01 |
Model | Template_relevance |
Template Set | Pistachio/Bkms_metabolic/Pistachio_ringbreaker/Reaxys/Reaxys_biocatalysis |
Top-N result to add to graph | 6 |
Feasible Synthetic Routes
Disclaimer and Information on In-Vitro Research Products
Please be aware that all articles and product information presented on BenchChem are intended solely for informational purposes. The products available for purchase on BenchChem are specifically designed for in-vitro studies, which are conducted outside of living organisms. In-vitro studies, derived from the Latin term "in glass," involve experiments performed in controlled laboratory settings using cells or tissues. It is important to note that these products are not categorized as medicines or drugs, and they have not received approval from the FDA for the prevention, treatment, or cure of any medical condition, ailment, or disease. We must emphasize that any form of bodily introduction of these products into humans or animals is strictly prohibited by law. It is essential to adhere to these guidelines to ensure compliance with legal and ethical standards in research and experimentation.