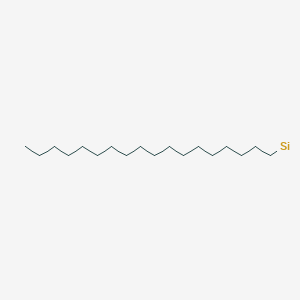
Octadecylsilane
Overview
Description
Tifacogin is a recombinant form of tissue factor pathway inhibitor, a protein that plays a crucial role in regulating blood coagulation. It has been investigated primarily for its potential therapeutic use in conditions such as severe sepsis and community-acquired pneumonia .
Mechanism of Action
Target of Action
Octadecylsilane, also known as Silane, octadecyl-, is an organosilicon compound that consists of a long-chain hydrocarbon (18 carbons) bonded to a silicon atom through a carbon-silicon bond . It is primarily targeted towards non-polar organic compounds due to its high surface activity .
Mode of Action
This compound interacts with its targets through a process known as reversed-phase binding. This nonpolar, nonendcapped sorbent provides reversed-phase binding of hydrophobic compounds . In addition, polar interactions are associated with the surface silanol groups . This functionality results in enhanced retention of basic compounds compared with the corresponding SampliQ C18 EC (endcapped) sorbent .
Biochemical Pathways
It is known that this compound can be used as a modifying agent for montmorillonite clay nanoparticles, which are used as a filler material in the synthesis of poly (methyl methacrylate) (pmma)/silylated montmorillonite composites .
Pharmacokinetics
It is known that the octanol/water partition coefficient p (log p) is a hydrophobicity index and is one of the determining factors for the pharmacokinetics of orally administered substances because it influences membrane permeability .
Result of Action
It is known that this compound provides excellent reproducibility and high recoveries in applications for neutral, weakly acidic, and basic compounds .
Action Environment
The action of this compound can be influenced by environmental factors. For example, the stationary phase in reversed-phase chromatography is usually quite hydrophobic, and these oil-like phases tend to minimize their surface energy through a tendency to self-associate . This can lead to a phenomenon known as phase collapse, where the stationary phase tends to collapse onto itself in a low-energy conformation .
Biochemical Analysis
Biochemical Properties
Octadecylsilane plays a significant role in biochemical reactions, particularly in the modification of silica surfaces. The compound interacts with silanol groups (Si–OH) on silica through chemical reactions, forming covalent bonds that enhance the hydrophobicity or hydrophilicity of the surface . This modification is crucial for applications such as incorporating silica particles into polymeric materials or increasing the hydrolytic stability of silica used in membranes . This compound also interacts with various biomolecules, including proteins, lipids, and nucleic acids, through hydrophobic interactions, which can influence the orientation and conformation of these biomolecules on modified surfaces .
Cellular Effects
This compound affects various types of cells and cellular processes. It influences cell function by modifying the surface properties of materials that cells interact with. For instance, the hydrophobic surface created by this compound can affect cell adhesion, proliferation, and differentiation . Additionally, the compound can impact cell signaling pathways, gene expression, and cellular metabolism by altering the microenvironment of cells . These effects are particularly relevant in the context of biosensors and biomaterials, where the interaction between cells and modified surfaces is critical for functionality.
Molecular Mechanism
The molecular mechanism of action of this compound involves its ability to form covalent bonds with silanol groups on silica surfaces, resulting in a modified surface with enhanced hydrophobicity . This modification can influence the binding interactions of biomolecules, such as proteins and lipids, with the surface. The hydrophobic interactions between this compound and biomolecules can lead to changes in the conformation and orientation of these biomolecules, affecting their biological activity . Additionally, this compound can modulate enzyme activity by altering the microenvironment and surface properties of the enzyme’s substrate .
Temporal Effects in Laboratory Settings
In laboratory settings, the effects of this compound can change over time due to its stability and degradation properties. The compound is generally stable under standard laboratory conditions, but its long-term effects on cellular function can vary depending on the specific application and environmental conditions . For instance, the hydrophobic surface created by this compound may undergo changes in wettability over time, which can influence the interaction between cells and the modified surface . Long-term studies have shown that the stability of this compound-modified surfaces can be maintained with proper storage and handling .
Dosage Effects in Animal Models
The effects of this compound vary with different dosages in animal models. At low doses, the compound is generally well-tolerated and can enhance the hydrophobicity of surfaces without causing significant adverse effects . At high doses, this compound may exhibit toxic effects, including irritation and inflammation, particularly in the respiratory system . Threshold effects have been observed, where the compound’s impact on cellular function and tissue integrity becomes more pronounced at higher concentrations .
Metabolic Pathways
This compound is involved in metabolic pathways related to its role as a surface modifier. The compound interacts with enzymes and cofactors that facilitate the modification of silica surfaces, including silanol groups and silicon alkoxides . These interactions can influence metabolic flux and metabolite levels by altering the surface properties of materials used in biochemical reactions . Additionally, this compound can affect the metabolism of cells by modifying the microenvironment and surface properties of cell culture substrates .
Transport and Distribution
This compound is transported and distributed within cells and tissues primarily through hydrophobic interactions with cellular membranes . The compound can interact with transporters and binding proteins that facilitate its movement within cells and tissues . Once inside the cell, this compound can accumulate in specific compartments, such as the cytoplasm and cell membrane, where it can exert its effects on cellular function .
Subcellular Localization
The subcellular localization of this compound is primarily in the cytoplasm and cell membrane . The compound’s hydrophobic nature allows it to integrate into lipid bilayers and interact with membrane proteins . This localization can influence the activity and function of this compound by affecting its interactions with other biomolecules and cellular structures . Additionally, targeting signals and post-translational modifications may direct this compound to specific compartments or organelles within the cell .
Preparation Methods
Synthetic Routes and Reaction Conditions: Tifacogin is produced using recombinant DNA technology. The gene encoding tissue factor pathway inhibitor is inserted into a suitable expression vector, which is then introduced into a host cell line, typically Chinese hamster ovary cells. The cells are cultured under controlled conditions to express the protein, which is subsequently purified using chromatographic techniques .
Industrial Production Methods: The industrial production of tifacogin involves large-scale fermentation of the genetically modified cells. The process includes several steps:
Cell Culture: The host cells are grown in bioreactors with a nutrient-rich medium.
Protein Expression: Induction of protein expression through specific conditions.
Harvesting: The cells are harvested, and the protein is extracted.
Purification: The protein undergoes multiple purification steps, including affinity chromatography and ion-exchange chromatography, to achieve high purity
Chemical Reactions Analysis
Types of Reactions: Tifacogin, being a protein, primarily undergoes biochemical reactions rather than traditional chemical reactions. These include:
Proteolysis: Breakdown of the protein by proteases.
Glycosylation: Addition of carbohydrate groups to the protein.
Common Reagents and Conditions:
Proteolysis: Enzymes such as trypsin or pepsin.
Glycosylation: Enzymes like glycosyltransferases.
Oxidation: Reactive oxygen species or specific oxidizing agents
Major Products Formed:
Proteolysis: Smaller peptide fragments.
Glycosylation: Glycoproteins with altered functional properties.
Oxidation: Oxidized protein forms with potential changes in activity
Scientific Research Applications
Tifacogin has been extensively studied for its potential therapeutic applications:
Severe Sepsis: Investigated for its ability to inhibit coagulation and reduce mortality in patients with severe sepsis
Community-Acquired Pneumonia: Studied as an adjunct therapy to improve outcomes in patients with severe pneumonia.
Anti-Inflammatory Effects: Explored for its anti-inflammatory properties in various inflammatory conditions.
Comparison with Similar Compounds
Recombinant Tissue Plasminogen Activator: Used for thrombolysis in acute myocardial infarction and stroke.
Antithrombin III: A natural anticoagulant used in hereditary antithrombin deficiency.
Comparison:
Uniqueness: Tifacogin specifically targets the tissue factor pathway, whereas recombinant tissue plasminogen activator and antithrombin III have broader anticoagulant effects.
Tifacogin’s targeted mechanism and potential therapeutic applications make it a unique and valuable compound in the field of medicine.
Properties
InChI |
InChI=1S/C18H37Si/c1-2-3-4-5-6-7-8-9-10-11-12-13-14-15-16-17-18-19/h2-18H2,1H3 | |
---|---|---|
Source | PubChem | |
URL | https://pubchem.ncbi.nlm.nih.gov | |
Description | Data deposited in or computed by PubChem | |
InChI Key |
FUESFIVIIFEDFI-UHFFFAOYSA-N | |
Source | PubChem | |
URL | https://pubchem.ncbi.nlm.nih.gov | |
Description | Data deposited in or computed by PubChem | |
Canonical SMILES |
CCCCCCCCCCCCCCCCCC[Si] | |
Source | PubChem | |
URL | https://pubchem.ncbi.nlm.nih.gov | |
Description | Data deposited in or computed by PubChem | |
Molecular Formula |
C18H37Si | |
Source | PubChem | |
URL | https://pubchem.ncbi.nlm.nih.gov | |
Description | Data deposited in or computed by PubChem | |
DSSTOX Substance ID |
DTXSID20885058 | |
Record name | Silane, octadecyl- | |
Source | EPA DSSTox | |
URL | https://comptox.epa.gov/dashboard/DTXSID20885058 | |
Description | DSSTox provides a high quality public chemistry resource for supporting improved predictive toxicology. | |
Molecular Weight |
281.6 g/mol | |
Source | PubChem | |
URL | https://pubchem.ncbi.nlm.nih.gov | |
Description | Data deposited in or computed by PubChem | |
CAS No. |
18623-11-5 | |
Record name | Octadecylsilane | |
Source | ChemIDplus | |
URL | https://pubchem.ncbi.nlm.nih.gov/substance/?source=chemidplus&sourceid=0018623115 | |
Description | ChemIDplus is a free, web search system that provides access to the structure and nomenclature authority files used for the identification of chemical substances cited in National Library of Medicine (NLM) databases, including the TOXNET system. | |
Record name | Silane, octadecyl- | |
Source | EPA Chemicals under the TSCA | |
URL | https://www.epa.gov/chemicals-under-tsca | |
Description | EPA Chemicals under the Toxic Substances Control Act (TSCA) collection contains information on chemicals and their regulations under TSCA, including non-confidential content from the TSCA Chemical Substance Inventory and Chemical Data Reporting. | |
Record name | Silane, octadecyl- | |
Source | EPA DSSTox | |
URL | https://comptox.epa.gov/dashboard/DTXSID20885058 | |
Description | DSSTox provides a high quality public chemistry resource for supporting improved predictive toxicology. | |
Record name | Octadecylsilane | |
Source | European Chemicals Agency (ECHA) | |
URL | https://echa.europa.eu/substance-information/-/substanceinfo/100.038.579 | |
Description | The European Chemicals Agency (ECHA) is an agency of the European Union which is the driving force among regulatory authorities in implementing the EU's groundbreaking chemicals legislation for the benefit of human health and the environment as well as for innovation and competitiveness. | |
Explanation | Use of the information, documents and data from the ECHA website is subject to the terms and conditions of this Legal Notice, and subject to other binding limitations provided for under applicable law, the information, documents and data made available on the ECHA website may be reproduced, distributed and/or used, totally or in part, for non-commercial purposes provided that ECHA is acknowledged as the source: "Source: European Chemicals Agency, http://echa.europa.eu/". Such acknowledgement must be included in each copy of the material. ECHA permits and encourages organisations and individuals to create links to the ECHA website under the following cumulative conditions: Links can only be made to webpages that provide a link to the Legal Notice page. | |
Record name | OCTADECYLSILANE | |
Source | FDA Global Substance Registration System (GSRS) | |
URL | https://gsrs.ncats.nih.gov/ginas/app/beta/substances/62PU8XK4BV | |
Description | The FDA Global Substance Registration System (GSRS) enables the efficient and accurate exchange of information on what substances are in regulated products. Instead of relying on names, which vary across regulatory domains, countries, and regions, the GSRS knowledge base makes it possible for substances to be defined by standardized, scientific descriptions. | |
Explanation | Unless otherwise noted, the contents of the FDA website (www.fda.gov), both text and graphics, are not copyrighted. They are in the public domain and may be republished, reprinted and otherwise used freely by anyone without the need to obtain permission from FDA. Credit to the U.S. Food and Drug Administration as the source is appreciated but not required. | |
Retrosynthesis Analysis
AI-Powered Synthesis Planning: Our tool employs the Template_relevance Pistachio, Template_relevance Bkms_metabolic, Template_relevance Pistachio_ringbreaker, Template_relevance Reaxys, Template_relevance Reaxys_biocatalysis model, leveraging a vast database of chemical reactions to predict feasible synthetic routes.
One-Step Synthesis Focus: Specifically designed for one-step synthesis, it provides concise and direct routes for your target compounds, streamlining the synthesis process.
Accurate Predictions: Utilizing the extensive PISTACHIO, BKMS_METABOLIC, PISTACHIO_RINGBREAKER, REAXYS, REAXYS_BIOCATALYSIS database, our tool offers high-accuracy predictions, reflecting the latest in chemical research and data.
Strategy Settings
Precursor scoring | Relevance Heuristic |
---|---|
Min. plausibility | 0.01 |
Model | Template_relevance |
Template Set | Pistachio/Bkms_metabolic/Pistachio_ringbreaker/Reaxys/Reaxys_biocatalysis |
Top-N result to add to graph | 6 |
Feasible Synthetic Routes
Q1: What is the molecular formula and weight of octadecylsilane?
A1: While the provided research articles don't explicitly state the molecular formula and weight of this compound, it's essential to clarify that the term "this compound" generally refers to a family of compounds with an 18-carbon alkyl chain (octadecyl) bonded to a silicon atom. The specific formula and weight depend on the substituents attached to the silicon atom. For example, octadecyltrichlorosilane, a common precursor for ODS stationary phases, has the formula CH3(CH2)17SiCl3 and a molecular weight of 387.8 g/mol.
Q2: How can Raman spectroscopy be used to characterize this compound stationary phases?
A2: Raman spectroscopy provides insights into the alkyl chain conformation of ODS stationary phases. Spectral data, particularly in the ν(C-C) and ν(C-H) regions, help assess the conformational order and its dependence on factors like temperature, surface coverage, and solvent environment [, , , , , , ]. This information is crucial for understanding the separation mechanisms and selectivity of ODS-based chromatographic columns.
Q3: How stable are this compound stationary phases under different conditions?
A3: The stability of ODS stationary phases depends on factors like the type of silica substrate, bonding density, and eluent composition. Research indicates that multifunctionally bonded ODS phases generally exhibit higher resistance to ligand stripping, especially in high pH eluents, compared to monofunctionally modified phases [].
Q4: What role does the silica substrate play in the stability of this compound stationary phases?
A4: The rigidity of the silica substrate influences the stability of the bonded phase, especially for monofunctionally modified ODS []. Using multifunctional silanes for modification can mitigate the influence of substrate characteristics on the stability compared to monofunctional ODS.
Q5: How does this compound function as a stationary phase in high-performance liquid chromatography (HPLC)?
A5: ODS is widely employed as a stationary phase in reversed-phase HPLC due to its hydrophobic nature. Analytes in the mobile phase interact with the nonpolar ODS chains through hydrophobic interactions, leading to their separation based on their relative hydrophobicity [, , , , , , , ].
Q6: What are the advantages of using this compound functionalized graphene oxide/silica composite as a stationary phase in HPLC?
A6: Incorporating graphene oxide (GO) into the ODS/silica stationary phase introduces π-π interactions between the analyte and the stationary phase, in addition to the typical hydrophobic interactions observed with traditional C18 columns. This additional interaction mechanism can enhance the separation of certain analytes, particularly those with aromatic rings [].
Q7: How do different mobile phase solvents affect the performance of this compound stationary phases?
A7: The type of solvent used in the mobile phase significantly influences the conformational order and solvation of the ODS stationary phase [, ]. Polar, hydrogen-bonding solvents tend to induce rotational ordering in high-density ODS phases, while hydrophobic solvents lead to greater disorder. Understanding these interactions is crucial for optimizing separation efficiency and selectivity in HPLC.
Q8: Can this compound be used for the separation of specific compounds or classes of compounds?
A8: Yes, ODS stationary phases are versatile and used for separating a wide range of compounds, including alkylbenzenes, polycyclic aromatic hydrocarbons, amines, phenolic compounds, triglycerides, fat-soluble vitamins, pesticides, and pharmaceuticals [, , , , , , , ]. The choice of mobile phase composition, temperature, and flow rate can be adjusted to optimize the separation of specific analytes.
Q9: How does the surface coverage of this compound on the silica support affect its chromatographic performance?
A9: Higher surface coverage of ODS generally leads to increased alkyl chain order and enhances shape selectivity in chromatographic separations [, , , ]. High-density ODS phases are particularly useful for separating structurally similar compounds that differ slightly in their shape or size.
Q10: What analytical techniques are commonly employed to quantify this compound in various matrices?
A10: While the provided research mainly focuses on the characterization and application of ODS-modified materials, techniques like elemental analysis and solid-state NMR can be used to quantify ODS loading on silica supports []. In the context of chromatographic applications, various detectors are employed depending on the analyte being analyzed, including UV detectors, mass spectrometers, and fluorescence detectors [, , , , ].
Q11: How does this compound contribute to the creation of optically anisotropic thin films?
A11: ODS, when combined with perylenesilane at liquid-liquid interfaces, can be used to create optically anisotropic 2D silica films []. These films exhibit varying wetting behaviors depending on the ODS concentration, highlighting the potential for developing sensitive and smart coatings.
Q12: What is the role of this compound in controlling the growth of organic semiconductors in thin-film applications?
A12: The density of the terminal methyl groups in ODS monolayers plays a crucial role in directing the growth of organic semiconductors like pentacene []. Crystalline, densely packed ODS layers promote two-dimensional pentacene growth, favorable for charge transport in organic thin-film transistors. In contrast, amorphous ODS layers lead to less desirable three-dimensional growth.
Q13: How is this compound used in the development of superhydrophobic surfaces?
A13: Polymerized n-octadecylsilane with micronano hierarchical structures created on quartz crystal microbalance (QCM) surfaces exhibits superhydrophobic properties []. These surfaces show promise as sensing materials for detecting volatile organic compounds, demonstrating the potential of ODS in sensor technology.
Disclaimer and Information on In-Vitro Research Products
Please be aware that all articles and product information presented on BenchChem are intended solely for informational purposes. The products available for purchase on BenchChem are specifically designed for in-vitro studies, which are conducted outside of living organisms. In-vitro studies, derived from the Latin term "in glass," involve experiments performed in controlled laboratory settings using cells or tissues. It is important to note that these products are not categorized as medicines or drugs, and they have not received approval from the FDA for the prevention, treatment, or cure of any medical condition, ailment, or disease. We must emphasize that any form of bodily introduction of these products into humans or animals is strictly prohibited by law. It is essential to adhere to these guidelines to ensure compliance with legal and ethical standards in research and experimentation.