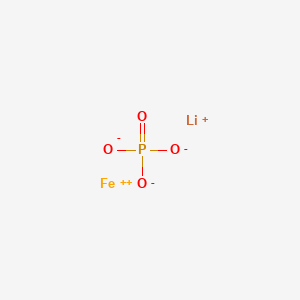
Lithium iron phosphate
- Click on QUICK INQUIRY to receive a quote from our team of experts.
- With the quality product at a COMPETITIVE price, you can focus more on your research.
Overview
Description
Lithium iron phosphate, also known as lithium ferro-phosphate, is an inorganic compound with the formula LiFePO₄. It is a gray, red-gray, brown, or black solid that is insoluble in water. This compound has gained significant attention as a component of this compound batteries, a type of lithium-ion battery. These batteries are widely used in power tools, electric vehicles, solar energy installations, and large grid-scale energy storage .
Synthetic Routes and Reaction Conditions:
Solid Phase Method: This includes high-temperature solid-phase reaction, carbothermal reduction, microwave synthesis, and mechanical alloying.
Liquid Phase Method: This involves processes such as hydrothermal synthesis and sol-gel methods. These methods typically offer better control over particle size and morphology.
Industrial Production Methods:
Grinding and Mixing: Lithium carbonate, sucrose, and pure water are mixed and ground.
High-Temperature Synthesis: The mixture is heated to high temperatures to facilitate the reaction and form this compound.
Types of Reactions:
Oxidation and Reduction: this compound undergoes reversible oxidation and reduction reactions, which are crucial for its function in batteries.
Substitution Reactions: Doping with other metals such as manganese, cobalt, or nickel can enhance the electrochemical properties of this compound.
Common Reagents and Conditions:
Oxidation: this compound can be oxidized using strong oxidizing agents under controlled conditions.
Reduction: Reduction typically involves the use of reducing agents such as hydrogen or carbon monoxide at elevated temperatures.
Major Products:
- The primary product of these reactions is this compound itself, which can be further processed or used directly in battery applications.
Chemistry:
- This compound is extensively studied for its use as a cathode material in lithium-ion batteries due to its high theoretical capacity, stability, and safety .
Biology and Medicine:
- While its primary applications are in energy storage, research is ongoing into its potential uses in biomedical devices and other biological applications.
Industry:
- This compound batteries are used in electric vehicles, renewable energy storage systems, and portable electronic devices. Their safety and long lifespan make them ideal for these applications .
Mechanism of Action
Target of Action
Lithium Iron Phosphate (LiFePO4), also known as LFP, is an inorganic compound primarily used as a component in this compound batteries . The primary targets of LiFePO4 are the cathode and anode in a battery, where it facilitates the movement of lithium ions during the charging and discharging process .
Mode of Action
The operation of a LiFePO4 battery involves the movement of lithium ions between the cathode and the anode during the charging and discharging process . This movement allows the battery to efficiently store and release electrical energy . During discharge, lithium ions move from the anode to the cathode through the electrolyte, while electrons flow through the external circuit, creating an electrical current . At the cathode, the lithium ions react with the LiFePO4 material, releasing electrons and forming Li3PO4 .
Biochemical Pathways
The biochemical pathways of LiFePO4 involve the diffusion of lithium ions in the crystal lattices of LiFePO4 . Surface decoration, nanocrystallization, and lattice substitution (doping) are modification approaches widely employed to promote the conductivity of electrons and the diffusion of lithium ions in the crystal lattices of LiFePO4 .
Pharmacokinetics
While the term “pharmacokinetics” is typically used in the context of drug metabolism, in the case of LiFePO4, we can consider its analogous process in terms of its Absorption, Distribution, Metabolism, and Excretion (ADME) within a battery system. The “absorption” and “distribution” occur when lithium ions move from the anode to the cathode during the discharging process . The “metabolism” happens when lithium ions react with the LiFePO4 material at the cathode . The “excretion” is analogous to the charging process, where lithium ions move from the cathode back to the anode .
Result of Action
The result of LiFePO4’s action is the efficient storage and release of electrical energy in a battery system . This allows for longer usage times, reducing the need for frequent recharging and keeping devices powered for longer .
Action Environment
The action of LiFePO4 can be influenced by various environmental factors. For instance, the ordering of LFP and the carbon additive particles facilitates the formation of evenly distributed pores owing to their distinct magnetic properties, which significantly decreases the ionic resistance of the LFP electrode . This modulation of pores and active materials enhances the lithium-ion conduction in the magnetically ordered LFP electrode . Furthermore, the environmental impact of LiFePO4 batteries has been studied, showing that electricity consumption during the manufacture and installation process is the greatest contributor to climate change .
Comparison with Similar Compounds
- Lithium cobalt oxide (LiCoO₂)
- Lithium manganese oxide (LiMn₂O₄)
- Lithium nickel oxide (LiNiO₂)
Comparison:
- Energy Density: Lithium cobalt oxide and lithium nickel oxide have higher energy densities compared to lithium iron phosphate, making them suitable for applications where space and weight are critical .
- Safety: this compound is safer and less prone to overheating and thermal runaway compared to lithium cobalt oxide and lithium nickel oxide .
- Cost: this compound is generally less expensive due to the abundance of iron and phosphate compared to cobalt and nickel .
This compound stands out due to its balance of safety, cost, and performance, making it a preferred choice for many applications despite its lower energy density.
Properties
CAS No. |
15365-14-7 |
---|---|
Molecular Formula |
FeH3LiO4P |
Molecular Weight |
160.8 g/mol |
IUPAC Name |
lithium;iron(2+);phosphate |
InChI |
InChI=1S/Fe.Li.H3O4P/c;;1-5(2,3)4/h;;(H3,1,2,3,4) |
InChI Key |
ZSYNKHJUSDFTCQ-UHFFFAOYSA-N |
SMILES |
[Li+].[O-]P(=O)([O-])[O-].[Fe+2] |
Canonical SMILES |
[Li].OP(=O)(O)O.[Fe] |
Key on ui other cas no. |
15365-14-7 |
Origin of Product |
United States |
Retrosynthesis Analysis
AI-Powered Synthesis Planning: Our tool employs the Template_relevance Pistachio, Template_relevance Bkms_metabolic, Template_relevance Pistachio_ringbreaker, Template_relevance Reaxys, Template_relevance Reaxys_biocatalysis model, leveraging a vast database of chemical reactions to predict feasible synthetic routes.
One-Step Synthesis Focus: Specifically designed for one-step synthesis, it provides concise and direct routes for your target compounds, streamlining the synthesis process.
Accurate Predictions: Utilizing the extensive PISTACHIO, BKMS_METABOLIC, PISTACHIO_RINGBREAKER, REAXYS, REAXYS_BIOCATALYSIS database, our tool offers high-accuracy predictions, reflecting the latest in chemical research and data.
Strategy Settings
Precursor scoring | Relevance Heuristic |
---|---|
Min. plausibility | 0.01 |
Model | Template_relevance |
Template Set | Pistachio/Bkms_metabolic/Pistachio_ringbreaker/Reaxys/Reaxys_biocatalysis |
Top-N result to add to graph | 6 |
Feasible Synthetic Routes
Disclaimer and Information on In-Vitro Research Products
Please be aware that all articles and product information presented on BenchChem are intended solely for informational purposes. The products available for purchase on BenchChem are specifically designed for in-vitro studies, which are conducted outside of living organisms. In-vitro studies, derived from the Latin term "in glass," involve experiments performed in controlled laboratory settings using cells or tissues. It is important to note that these products are not categorized as medicines or drugs, and they have not received approval from the FDA for the prevention, treatment, or cure of any medical condition, ailment, or disease. We must emphasize that any form of bodily introduction of these products into humans or animals is strictly prohibited by law. It is essential to adhere to these guidelines to ensure compliance with legal and ethical standards in research and experimentation.