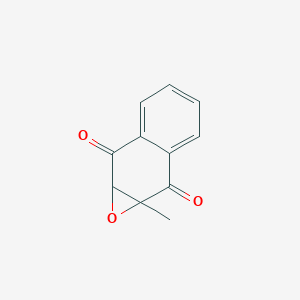
Menadione epoxide
Overview
Description
Mechanism of Action
Target of Action
Menadione, also known as Vitamin K3, is a synthetic naphthoquinone without the isoprenoid side chain and biological activity . It can be converted to active vitamin K2, menaquinone, after alkylation in vivo . The primary targets of Menadione are the vitamin K-dependent coagulation factors II (prothrombin), VII (proconvertin), IX (Christmas factor), X (Stuart factor), protein C, protein S . These proteins play a crucial role in the normal clotting of blood .
Mode of Action
Menadione is involved as a cofactor in the posttranslational gamma-carboxylation of glutamic acid residues of certain proteins in the body . This interaction with its targets leads to changes in the coagulation factors, enabling them to bind calcium ions, which is necessary for their activity in the coagulation cascade . In the case of Menadione epoxide, it is formed through oxidation reactions in vivo, that occur in protein processes dependent on vitamin K .
Biochemical Pathways
Menadione is converted to vitamin K2 (specifically, MK-4) by the prenyltransferase action of vertebrate UBIAD1 . This reaction requires the hydroquinone (reduced) form of K3, menadiol, produced by NQO1 . Menadione is also a circulating form of vitamin K, produced in small amounts (1–5%) after intestinal absorption of K1 and K2 . This circulation explains the uneven tissue distribution of MK-4, especially since menadione can penetrate the blood–brain barrier .
Pharmacokinetics
Menadione (Vitamin K3) is a fat-soluble vitamin precursor that is converted into menaquinone in the liver . Vitamin K1 and K2 are the naturally occurring types of vitamin K . The former, which is also known as phylloquinone, is synthesized by plants and can be found in such foods as spinach, broccoli, lettuce, and soybeans . The latter, sometimes alternatively referred to as menaquinone, is primarily produced by bacteria in the anterior part of the gut and the intestines .
Result of Action
The result of Menadione’s action is the activation of the vitamin K-dependent coagulation factors, which play a crucial role in the normal clotting of blood . An overdose of Vitamin K3 can be detrimental, especially to infants that may easily suffer from extensive hemorrhaging . Newborns that are administered too great a dosage of vitamin K3 can suffer from kernicterus, a form of severe brain damage that may produce decreased movement, loss of appetite, seizures, deafness, mental retardation, and even death .
Action Environment
A variety of studies has shown a wide range of biological activities of Menadione, such as anticancer, antibacterial, antifungal, antimalarial, antichagasic, and anthelmintic effects . In these cases, the redox cycle is influenced by environmental factors .
Biochemical Analysis
Biochemical Properties
Menadione epoxide, like its parent compound Menadione, plays a role in biochemical reactions. Menadione is involved as a cofactor in the posttranslational gamma-carboxylation of glutamic acid residues of certain proteins in the body
Cellular Effects
The cellular effects of this compound are not well-documented. Menadione, from which this compound is derived, is known to have significant effects on cells. Menadione is converted into menaquinone in the liver . While a vitamin K deficiency can be dangerous, especially to infants that may easily suffer from extensive hemorrhaging, an overdose can be as equally detrimental .
Molecular Mechanism
Menadione, its parent compound, is involved as a cofactor in the posttranslational gamma-carboxylation of glutamic acid residues of certain proteins in the body . This process is facilitated by the vitamin K cycle involving γ-carboxyglutamyl carboxylase, vitamin K epoxide reductase, and ferroptosis suppressor protein-1 .
Temporal Effects in Laboratory Settings
The temporal effects of this compound in laboratory settings are not well-documented. Menadione, its parent compound, has been studied extensively. Menadione is a synthetic naphthoquinone that can be converted to active vitamin K2, menaquinone, after alkylation in vivo .
Dosage Effects in Animal Models
The effects of this compound dosage in animal models are not well-documented. Menadione, its parent compound, has been studied. Menadione is a fat-soluble vitamin precursor that is converted into menaquinone in the liver . An overdose of Menadione can be detrimental, especially to infants .
Metabolic Pathways
Menadione, its parent compound, is known to be involved in the vitamin K cycle . This cycle involves the conversion of Menadione to vitamin K2 (specifically, MK-4) by the prenyltransferase action of vertebrate UBIAD1 .
Transport and Distribution
Menadione, its parent compound, is a fat-soluble vitamin precursor that is converted into menaquinone in the liver .
Subcellular Localization
Menadione, its parent compound, is known to be both cytosolic and peroxisomal in human hepatocytes and renal proximal tubules .
Preparation Methods
Synthetic Routes and Reaction Conditions: Menadione epoxide can be synthesized through the epoxidation of menadione. One common method involves the use of hydrogen peroxide as the oxidant in the presence of a catalyst. For instance, the zeolite imidazolate framework ZIF-8 has been shown to exhibit superior catalytic performance in the epoxidation of the electron-deficient carbon-carbon double bond in menadione using aqueous hydrogen peroxide .
Industrial Production Methods: While specific industrial production methods for this compound are not extensively documented, the general approach involves the use of heterogeneous catalysts and oxidizing agents to achieve efficient and scalable synthesis
Chemical Reactions Analysis
Types of Reactions: Menadione epoxide undergoes various chemical reactions, including:
Oxidation: this compound can be further oxidized to form different quinone derivatives.
Reduction: Reduction reactions can convert this compound back to menadione or other reduced forms.
Substitution: Nucleophilic substitution reactions can occur at the epoxide ring, leading to the formation of various substituted derivatives.
Common Reagents and Conditions:
Oxidation: Hydrogen peroxide is commonly used as an oxidant in the presence of catalysts like ZIF-8.
Reduction: Reducing agents such as sodium borohydride can be used to reduce this compound.
Substitution: Nucleophiles such as amines or thiols can react with the epoxide ring under mild conditions.
Major Products Formed:
Oxidation: Further oxidized quinone derivatives.
Reduction: Menadione and its reduced forms.
Substitution: Substituted naphthoquinone derivatives.
Scientific Research Applications
Menadione epoxide has several scientific research applications, including:
Chemistry: Used as a precursor for the synthesis of various quinone derivatives and as a model compound for studying redox reactions.
Biology: Investigated for its potential role in biological redox cycles and its interactions with biomolecules.
Medicine: Explored for its cytotoxic activity against cancer cells and its potential use in developing new anticancer drugs.
Industry: Utilized in the synthesis of bioactive compounds and as a catalyst in organic reactions.
Comparison with Similar Compounds
Menadione epoxide is unique due to its epoxide ring, which imparts distinct chemical reactivity compared to other naphthoquinones. Similar compounds include:
Menadione (2-methyl-1,4-naphthoquinone): The parent compound of this compound, used as a vitamin K precursor.
Phylloquinone (Vitamin K1): A naturally occurring form of vitamin K found in green plants.
Menaquinone (Vitamin K2): A group of compounds produced by bacteria in the gut and involved in bone and cardiovascular health.
This compound’s unique structure and reactivity make it a valuable compound for various scientific and industrial applications.
Properties
IUPAC Name |
1a-methyl-7aH-naphtho[2,3-b]oxirene-2,7-dione | |
---|---|---|
Source | PubChem | |
URL | https://pubchem.ncbi.nlm.nih.gov | |
Description | Data deposited in or computed by PubChem | |
InChI |
InChI=1S/C11H8O3/c1-11-9(13)7-5-3-2-4-6(7)8(12)10(11)14-11/h2-5,10H,1H3 | |
Source | PubChem | |
URL | https://pubchem.ncbi.nlm.nih.gov | |
Description | Data deposited in or computed by PubChem | |
InChI Key |
NNUKDUBCRRYXDC-UHFFFAOYSA-N | |
Source | PubChem | |
URL | https://pubchem.ncbi.nlm.nih.gov | |
Description | Data deposited in or computed by PubChem | |
Canonical SMILES |
CC12C(O1)C(=O)C3=CC=CC=C3C2=O | |
Source | PubChem | |
URL | https://pubchem.ncbi.nlm.nih.gov | |
Description | Data deposited in or computed by PubChem | |
Molecular Formula |
C11H8O3 | |
Source | PubChem | |
URL | https://pubchem.ncbi.nlm.nih.gov | |
Description | Data deposited in or computed by PubChem | |
DSSTOX Substance ID |
DTXSID80934995 | |
Record name | 1a-Methyl-1a,7a-dihydronaphtho[2,3-b]oxirene-2,7-dione | |
Source | EPA DSSTox | |
URL | https://comptox.epa.gov/dashboard/DTXSID80934995 | |
Description | DSSTox provides a high quality public chemistry resource for supporting improved predictive toxicology. | |
Molecular Weight |
188.18 g/mol | |
Source | PubChem | |
URL | https://pubchem.ncbi.nlm.nih.gov | |
Description | Data deposited in or computed by PubChem | |
CAS No. |
15448-59-6 | |
Record name | 2,3-Epoxy-2-methyl-1,4-naphthoquinone | |
Source | CAS Common Chemistry | |
URL | https://commonchemistry.cas.org/detail?cas_rn=15448-59-6 | |
Description | CAS Common Chemistry is an open community resource for accessing chemical information. Nearly 500,000 chemical substances from CAS REGISTRY cover areas of community interest, including common and frequently regulated chemicals, and those relevant to high school and undergraduate chemistry classes. This chemical information, curated by our expert scientists, is provided in alignment with our mission as a division of the American Chemical Society. | |
Explanation | The data from CAS Common Chemistry is provided under a CC-BY-NC 4.0 license, unless otherwise stated. | |
Record name | Menadione epoxide | |
Source | ChemIDplus | |
URL | https://pubchem.ncbi.nlm.nih.gov/substance/?source=chemidplus&sourceid=0015448596 | |
Description | ChemIDplus is a free, web search system that provides access to the structure and nomenclature authority files used for the identification of chemical substances cited in National Library of Medicine (NLM) databases, including the TOXNET system. | |
Record name | Menadione epoxide | |
Source | DTP/NCI | |
URL | https://dtp.cancer.gov/dtpstandard/servlet/dwindex?searchtype=NSC&outputformat=html&searchlist=65669 | |
Description | The NCI Development Therapeutics Program (DTP) provides services and resources to the academic and private-sector research communities worldwide to facilitate the discovery and development of new cancer therapeutic agents. | |
Explanation | Unless otherwise indicated, all text within NCI products is free of copyright and may be reused without our permission. Credit the National Cancer Institute as the source. | |
Record name | 1a-Methyl-1a,7a-dihydronaphtho[2,3-b]oxirene-2,7-dione | |
Source | EPA DSSTox | |
URL | https://comptox.epa.gov/dashboard/DTXSID80934995 | |
Description | DSSTox provides a high quality public chemistry resource for supporting improved predictive toxicology. | |
Synthesis routes and methods
Procedure details
Retrosynthesis Analysis
AI-Powered Synthesis Planning: Our tool employs the Template_relevance Pistachio, Template_relevance Bkms_metabolic, Template_relevance Pistachio_ringbreaker, Template_relevance Reaxys, Template_relevance Reaxys_biocatalysis model, leveraging a vast database of chemical reactions to predict feasible synthetic routes.
One-Step Synthesis Focus: Specifically designed for one-step synthesis, it provides concise and direct routes for your target compounds, streamlining the synthesis process.
Accurate Predictions: Utilizing the extensive PISTACHIO, BKMS_METABOLIC, PISTACHIO_RINGBREAKER, REAXYS, REAXYS_BIOCATALYSIS database, our tool offers high-accuracy predictions, reflecting the latest in chemical research and data.
Strategy Settings
Precursor scoring | Relevance Heuristic |
---|---|
Min. plausibility | 0.01 |
Model | Template_relevance |
Template Set | Pistachio/Bkms_metabolic/Pistachio_ringbreaker/Reaxys/Reaxys_biocatalysis |
Top-N result to add to graph | 6 |
Feasible Synthetic Routes
Disclaimer and Information on In-Vitro Research Products
Please be aware that all articles and product information presented on BenchChem are intended solely for informational purposes. The products available for purchase on BenchChem are specifically designed for in-vitro studies, which are conducted outside of living organisms. In-vitro studies, derived from the Latin term "in glass," involve experiments performed in controlled laboratory settings using cells or tissues. It is important to note that these products are not categorized as medicines or drugs, and they have not received approval from the FDA for the prevention, treatment, or cure of any medical condition, ailment, or disease. We must emphasize that any form of bodily introduction of these products into humans or animals is strictly prohibited by law. It is essential to adhere to these guidelines to ensure compliance with legal and ethical standards in research and experimentation.