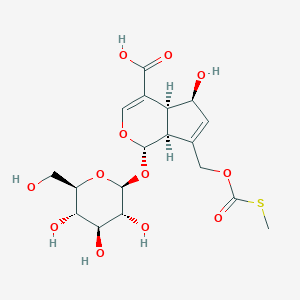
Paederosidic acid
Overview
Description
Iron glycinate, also known as ferrous bisglycinate, is a chelated form of iron where iron is bound to the amino acid glycine. This compound is known for its high bioavailability and minimal gastrointestinal side effects compared to other iron supplements. It is commonly used to treat and prevent iron deficiency anemia.
Mechanism of Action
Target of Action
Paederosidic acid, an iridoid glycoside isolated from Paederia scandens, has been found to interact with several targets. The primary target of this compound is the P2Y receptor (P2YR) . The activation of P2YR promotes osteoclast formation and causes neuropathic pain, exhibiting a possible link to osteoarthritis .
Mode of Action
This compound directly interacts with P2YR, enhancing the thermostability and decreasing the protease sensitivity of the target protein . This interaction significantly inhibits receptor activator for nuclear factor-κB ligand (RANKL)-mediated osteoclastogenesis .
Biochemical Pathways
The action of this compound affects several biochemical pathways. It induces mitochondria-mediated apoptosis in cancer cells . This process involves the up-regulation of caspase-3, caspase-8, caspase-9, Bid, Bax, and the down-regulation of Bcl-2, stimulating the release of Cyto-C from mitochondria .
Pharmacokinetics
The pharmacokinetics of this compound have been studied in rat plasma . The bioavailability (F) of this compound was found to be between 1.74% and 3.36% . .
Result of Action
This compound exhibits significant anti-cancer and anti-inflammatory activities . It inhibits lung cancer cells via inducing mitochondria-mediated apoptosis . In addition, it has been found to exert significant inhibitory effects on gastric cancer cell lines .
Biochemical Analysis
Biochemical Properties
Paederosidic acid plays a crucial role in biochemical reactions, particularly in inducing apoptosis in cancer cells. It interacts with several enzymes and proteins, including caspase-3, caspase-8, and caspase-9, which are key players in the apoptotic pathway . The compound upregulates pro-apoptotic proteins such as Bid and Bax while downregulating anti-apoptotic proteins like Bcl-2 . Additionally, this compound stimulates the release of cytochrome c from mitochondria, further promoting apoptosis .
Cellular Effects
This compound exerts significant effects on various cell types and cellular processes. In lung cancer cells, it induces mitochondria-mediated apoptosis, leading to cell death . The compound also affects cell signaling pathways by modulating the expression of genes involved in apoptosis and inflammation . In hepatocellular carcinoma cells, this compound inhibits cell growth, invasion, and angiogenesis while promoting apoptosis . These effects are dose-dependent and highlight the compound’s potential as an anticancer agent .
Molecular Mechanism
At the molecular level, this compound exerts its effects through several mechanisms. It binds to and activates caspases, leading to the cleavage of various substrates involved in apoptosis . The compound also inhibits the P2Y14 receptor, which is implicated in osteoclast formation and neuropathic pain . By downregulating the expression of NFAT2 and ATP6V0D2, this compound suppresses osteoclastogenesis and alleviates pain . These molecular interactions underscore the compound’s therapeutic potential in treating cancer and osteoarthritis .
Temporal Effects in Laboratory Settings
In laboratory settings, the effects of this compound change over time. The compound is stable at room temperature and exhibits long-term effects on cellular function when stored appropriately . In vitro studies have shown that this compound maintains its bioactivity for extended periods, with significant effects observed after 24 to 48 hours of treatment . In vivo studies indicate that the compound’s effects on cellular function, such as apoptosis and inflammation, persist over time, highlighting its potential for long-term therapeutic use .
Dosage Effects in Animal Models
The effects of this compound vary with different dosages in animal models. At lower doses, the compound exhibits significant antinociceptive and anti-inflammatory effects . At higher doses, this compound may cause adverse effects, including toxicity . Studies have shown that the compound’s therapeutic window is relatively wide, allowing for effective treatment at doses that minimize toxicity . These findings underscore the importance of dosage optimization in preclinical and clinical studies.
Metabolic Pathways
This compound is involved in several metabolic pathways, primarily those related to apoptosis and inflammation . The compound interacts with enzymes such as caspases and proteins involved in the mitochondrial apoptotic pathway . By modulating these pathways, this compound influences metabolic flux and metabolite levels, contributing to its bioactivity . These interactions highlight the compound’s potential as a therapeutic agent in diseases characterized by dysregulated apoptosis and inflammation.
Transport and Distribution
Within cells and tissues, this compound is transported and distributed through various mechanisms. The compound interacts with transporters and binding proteins that facilitate its uptake and localization . Once inside the cell, this compound accumulates in specific compartments, such as mitochondria, where it exerts its apoptotic effects . These transport and distribution mechanisms are crucial for the compound’s bioavailability and therapeutic efficacy.
Subcellular Localization
This compound is primarily localized in the mitochondria, where it induces apoptosis by modulating the mitochondrial membrane potential and promoting the release of cytochrome c . The compound’s subcellular localization is directed by targeting signals and post-translational modifications that ensure its delivery to the appropriate cellular compartments . This precise localization is essential for this compound’s bioactivity and therapeutic potential.
Preparation Methods
Synthetic Routes and Reaction Conditions: Iron glycinate is typically synthesized by reacting reduced iron with glycine in the presence of citric acid. The reaction is carried out at a controlled temperature to ensure the formation of the chelate. The resulting product is then spray-dried to obtain a fine, free-flowing powder .
Industrial Production Methods: In industrial settings, iron glycinate is produced by reacting iron powder with glycine at a temperature of around 50°C for 24 hours. Alternatively, it can be prepared by reacting glycine with ferric chloride and then adding sodium hydroxide to precipitate the iron glycinate .
Chemical Reactions Analysis
Types of Reactions: Iron glycinate undergoes various chemical reactions, including:
Oxidation: Iron in the glycinate complex can be oxidized from ferrous (Fe²⁺) to ferric (Fe³⁺) state.
Reduction: Ferric iron can be reduced back to ferrous iron under certain conditions.
Complexation: Iron glycinate can form complexes with other ligands, affecting its stability and reactivity
Common Reagents and Conditions:
Oxidation: Oxygen or hydrogen peroxide can be used as oxidizing agents.
Reduction: Reducing agents like ascorbic acid can be employed.
Complexation: Various ligands such as ethylenediaminetetraacetic acid (EDTA) can be used to study complex formation.
Major Products Formed:
Oxidation: Ferric glycinate.
Reduction: Ferrous glycinate.
Complexation: Various iron-ligand complexes depending on the ligands used
Scientific Research Applications
Iron glycinate has a wide range of applications in scientific research:
Chemistry: Used as a model compound to study iron coordination chemistry and complexation reactions.
Biology: Investigated for its role in iron metabolism and transport in biological systems.
Medicine: Widely used as an iron supplement to treat iron deficiency anemia due to its high bioavailability and minimal side effects
Industry: Employed in food fortification and as a nutrient supplement in various food products
Comparison with Similar Compounds
- Ferrous sulfate
- Ferrous fumarate
- Ferrous gluconate
- Ferric citrate
Comparison:
- Bioavailability: Iron glycinate has higher bioavailability compared to ferrous sulfate and ferrous fumarate.
- Side Effects: Iron glycinate causes fewer gastrointestinal side effects compared to other iron salts.
- Stability: The chelated form of iron glycinate is more stable and less affected by dietary inhibitors of iron absorption .
Iron glycinate stands out due to its superior absorption and minimal side effects, making it a preferred choice for iron supplementation.
Properties
IUPAC Name |
(1S,4aS,5S,7aS)-5-hydroxy-7-(methylsulfanylcarbonyloxymethyl)-1-[(2S,3R,4S,5S,6R)-3,4,5-trihydroxy-6-(hydroxymethyl)oxan-2-yl]oxy-1,4a,5,7a-tetrahydrocyclopenta[c]pyran-4-carboxylic acid | |
---|---|---|
Source | PubChem | |
URL | https://pubchem.ncbi.nlm.nih.gov | |
Description | Data deposited in or computed by PubChem | |
InChI |
InChI=1S/C18H24O12S/c1-31-18(26)28-4-6-2-8(20)11-7(15(24)25)5-27-16(10(6)11)30-17-14(23)13(22)12(21)9(3-19)29-17/h2,5,8-14,16-17,19-23H,3-4H2,1H3,(H,24,25)/t8-,9+,10+,11-,12+,13-,14+,16-,17-/m0/s1 | |
Source | PubChem | |
URL | https://pubchem.ncbi.nlm.nih.gov | |
Description | Data deposited in or computed by PubChem | |
InChI Key |
ICTKKPLVSHVNDV-FCVLBCLDSA-N | |
Source | PubChem | |
URL | https://pubchem.ncbi.nlm.nih.gov | |
Description | Data deposited in or computed by PubChem | |
Canonical SMILES |
CSC(=O)OCC1=CC(C2C1C(OC=C2C(=O)O)OC3C(C(C(C(O3)CO)O)O)O)O | |
Source | PubChem | |
URL | https://pubchem.ncbi.nlm.nih.gov | |
Description | Data deposited in or computed by PubChem | |
Isomeric SMILES |
CSC(=O)OCC1=C[C@@H]([C@H]2[C@@H]1[C@@H](OC=C2C(=O)O)O[C@H]3[C@@H]([C@H]([C@@H]([C@H](O3)CO)O)O)O)O | |
Source | PubChem | |
URL | https://pubchem.ncbi.nlm.nih.gov | |
Description | Data deposited in or computed by PubChem | |
Molecular Formula |
C18H24O12S | |
Source | PubChem | |
URL | https://pubchem.ncbi.nlm.nih.gov | |
Description | Data deposited in or computed by PubChem | |
Molecular Weight |
464.4 g/mol | |
Source | PubChem | |
URL | https://pubchem.ncbi.nlm.nih.gov | |
Description | Data deposited in or computed by PubChem | |
Retrosynthesis Analysis
AI-Powered Synthesis Planning: Our tool employs the Template_relevance Pistachio, Template_relevance Bkms_metabolic, Template_relevance Pistachio_ringbreaker, Template_relevance Reaxys, Template_relevance Reaxys_biocatalysis model, leveraging a vast database of chemical reactions to predict feasible synthetic routes.
One-Step Synthesis Focus: Specifically designed for one-step synthesis, it provides concise and direct routes for your target compounds, streamlining the synthesis process.
Accurate Predictions: Utilizing the extensive PISTACHIO, BKMS_METABOLIC, PISTACHIO_RINGBREAKER, REAXYS, REAXYS_BIOCATALYSIS database, our tool offers high-accuracy predictions, reflecting the latest in chemical research and data.
Strategy Settings
Precursor scoring | Relevance Heuristic |
---|---|
Min. plausibility | 0.01 |
Model | Template_relevance |
Template Set | Pistachio/Bkms_metabolic/Pistachio_ringbreaker/Reaxys/Reaxys_biocatalysis |
Top-N result to add to graph | 6 |
Feasible Synthetic Routes
Disclaimer and Information on In-Vitro Research Products
Please be aware that all articles and product information presented on BenchChem are intended solely for informational purposes. The products available for purchase on BenchChem are specifically designed for in-vitro studies, which are conducted outside of living organisms. In-vitro studies, derived from the Latin term "in glass," involve experiments performed in controlled laboratory settings using cells or tissues. It is important to note that these products are not categorized as medicines or drugs, and they have not received approval from the FDA for the prevention, treatment, or cure of any medical condition, ailment, or disease. We must emphasize that any form of bodily introduction of these products into humans or animals is strictly prohibited by law. It is essential to adhere to these guidelines to ensure compliance with legal and ethical standards in research and experimentation.