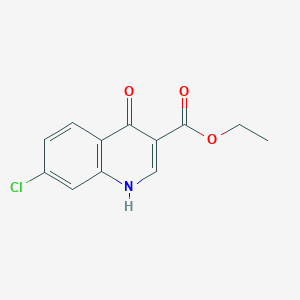
Ethyl 7-chloro-4-hydroxyquinoline-3-carboxylate
Overview
Description
Ethyl 7-chloro-4-hydroxyquinoline-3-carboxylate is an organic compound with the molecular formula C12H10ClNO3 It is a derivative of quinoline, a heterocyclic aromatic organic compound
Preparation Methods
Synthetic Routes and Reaction Conditions
Ethyl 7-chloro-4-hydroxyquinoline-3-carboxylate can be synthesized through several methods. One common approach involves the reaction of 7-chloro-4-hydroxyquinoline-3-carboxylic acid with ethanol in the presence of a dehydrating agent such as thionyl chloride or phosphorus oxychloride. The reaction typically occurs under reflux conditions, and the product is purified through recrystallization .
Industrial Production Methods
In industrial settings, the production of this compound often involves large-scale batch or continuous processes. The reaction conditions are optimized to ensure high yield and purity. Solvents such as dichloromethane or toluene may be used to facilitate the reaction, and the product is isolated through filtration and drying .
Chemical Reactions Analysis
Types of Reactions
Ethyl 7-chloro-4-hydroxyquinoline-3-carboxylate undergoes various chemical reactions, including:
Oxidation: The hydroxyl group can be oxidized to form a quinone derivative.
Reduction: The nitro group, if present, can be reduced to an amine.
Substitution: The chlorine atom can be substituted with other nucleophiles such as amines or thiols.
Common Reagents and Conditions
Oxidation: Reagents such as potassium permanganate or chromium trioxide are commonly used.
Reduction: Catalytic hydrogenation or metal hydrides like lithium aluminum hydride are employed.
Substitution: Nucleophiles such as sodium azide or thiourea can be used under basic conditions.
Major Products Formed
Oxidation: Quinone derivatives.
Reduction: Amino derivatives.
Substitution: Various substituted quinoline derivatives.
Scientific Research Applications
Ethyl 7-chloro-4-hydroxyquinoline-3-carboxylate has several scientific research applications:
Chemistry: Used as a building block for the synthesis of more complex molecules.
Biology: Investigated for its potential antimicrobial and antiviral properties.
Medicine: Explored as a potential therapeutic agent for various diseases.
Mechanism of Action
The mechanism of action of ethyl 7-chloro-4-hydroxyquinoline-3-carboxylate involves its interaction with specific molecular targets. It can inhibit the activity of certain enzymes or interfere with the replication of microbial DNA. The compound’s effects are mediated through pathways involving oxidative stress and disruption of cellular processes .
Comparison with Similar Compounds
Similar Compounds
Ethyl 7-chloro-6-fluoro-4-hydroxyquinoline-3-carboxylate: Similar structure with a fluorine atom instead of a hydrogen atom.
Ethyl 7-chloro-4-hydroxyquinoline-3-carboxylic acid: The carboxylate group is not esterified.
Ethyl 7-chloro-4-hydroxyquinoline-3-carboxamide: The carboxylate group is converted to an amide.
Uniqueness
Ethyl 7-chloro-4-hydroxyquinoline-3-carboxylate is unique due to its specific substitution pattern, which imparts distinct chemical and biological properties. The presence of the ethyl ester group enhances its solubility and bioavailability compared to its acid or amide counterparts .
Biological Activity
Ethyl 7-chloro-4-hydroxyquinoline-3-carboxylate is a compound of significant interest in medicinal chemistry due to its diverse biological activities. This article provides a comprehensive overview of its biological properties, mechanisms of action, and potential therapeutic applications, supported by relevant data and case studies.
Chemical Structure and Properties
This compound has the molecular formula and a molecular weight of approximately 269.66 g/mol. The compound features a quinoline ring system, which is known for its pharmacological significance.
Antimicrobial Activity
This compound has been studied for its antimicrobial properties . Research indicates that it exhibits activity against various bacterial strains, particularly Helicobacter pylori , which is associated with gastric ulcers and cancer. In a study, derivatives of this compound showed potent antimicrobial effects, with minimum inhibitory concentrations (MIC) as low as 25 μg/mL against H. pylori strains .
Compound | MIC (μg/mL) | Target |
---|---|---|
This compound | 25 | H. pylori |
Other derivatives | 10-50 | Various bacterial strains |
Anticancer Activity
The compound also demonstrates anticancer properties . In vitro studies have shown that it can induce cytotoxicity in several cancer cell lines, including breast (MCF-7) and cervical (HeLa) cancer cells. The mechanism appears to involve the inhibition of specific cellular pathways critical for tumor growth and survival .
Antioxidative Effects
Research has explored the antioxidative potential of this compound. It was found to exhibit prooxidative activity under certain conditions, suggesting that it may play a dual role in cellular oxidative stress modulation. This property could be beneficial in therapeutic contexts where oxidative stress is implicated .
The biological activity of this compound is attributed to its ability to interact with various molecular targets:
- Enzyme Inhibition : The compound inhibits enzymes involved in bacterial cell wall synthesis and cancer cell proliferation.
- Reactive Oxygen Species (ROS) Modulation : It influences ROS levels, potentially leading to oxidative damage in susceptible cells.
- Cell Cycle Arrest : In cancer cells, it may induce cell cycle arrest, preventing further proliferation.
Case Studies
-
Antimicrobial Efficacy Against H. pylori :
A study conducted on the effects of this compound revealed significant inhibition of H. pylori growth in laboratory settings, suggesting its potential as a therapeutic agent for gastric infections . -
Cytotoxicity in Cancer Cells :
In vitro assays demonstrated that this compound effectively reduced cell viability in MCF-7 and HeLa cell lines, indicating its potential use in cancer therapy .
Properties
IUPAC Name |
ethyl 7-chloro-4-oxo-1H-quinoline-3-carboxylate | |
---|---|---|
Source | PubChem | |
URL | https://pubchem.ncbi.nlm.nih.gov | |
Description | Data deposited in or computed by PubChem | |
InChI |
InChI=1S/C12H10ClNO3/c1-2-17-12(16)9-6-14-10-5-7(13)3-4-8(10)11(9)15/h3-6H,2H2,1H3,(H,14,15) | |
Source | PubChem | |
URL | https://pubchem.ncbi.nlm.nih.gov | |
Description | Data deposited in or computed by PubChem | |
InChI Key |
XWMCHSWUDMFGSW-UHFFFAOYSA-N | |
Source | PubChem | |
URL | https://pubchem.ncbi.nlm.nih.gov | |
Description | Data deposited in or computed by PubChem | |
Canonical SMILES |
CCOC(=O)C1=CNC2=C(C1=O)C=CC(=C2)Cl | |
Source | PubChem | |
URL | https://pubchem.ncbi.nlm.nih.gov | |
Description | Data deposited in or computed by PubChem | |
Molecular Formula |
C12H10ClNO3 | |
Source | PubChem | |
URL | https://pubchem.ncbi.nlm.nih.gov | |
Description | Data deposited in or computed by PubChem | |
DSSTOX Substance ID |
DTXSID80323108 | |
Record name | Ethyl 7-chloro-4-oxo-1,4-dihydroquinoline-3-carboxylate | |
Source | EPA DSSTox | |
URL | https://comptox.epa.gov/dashboard/DTXSID80323108 | |
Description | DSSTox provides a high quality public chemistry resource for supporting improved predictive toxicology. | |
Molecular Weight |
251.66 g/mol | |
Source | PubChem | |
URL | https://pubchem.ncbi.nlm.nih.gov | |
Description | Data deposited in or computed by PubChem | |
CAS No. |
16600-22-9 | |
Record name | 16600-22-9 | |
Source | DTP/NCI | |
URL | https://dtp.cancer.gov/dtpstandard/servlet/dwindex?searchtype=NSC&outputformat=html&searchlist=403048 | |
Description | The NCI Development Therapeutics Program (DTP) provides services and resources to the academic and private-sector research communities worldwide to facilitate the discovery and development of new cancer therapeutic agents. | |
Explanation | Unless otherwise indicated, all text within NCI products is free of copyright and may be reused without our permission. Credit the National Cancer Institute as the source. | |
Record name | Ethyl 7-chloro-4-oxo-1,4-dihydroquinoline-3-carboxylate | |
Source | EPA DSSTox | |
URL | https://comptox.epa.gov/dashboard/DTXSID80323108 | |
Description | DSSTox provides a high quality public chemistry resource for supporting improved predictive toxicology. | |
Synthesis routes and methods
Procedure details
Retrosynthesis Analysis
AI-Powered Synthesis Planning: Our tool employs the Template_relevance Pistachio, Template_relevance Bkms_metabolic, Template_relevance Pistachio_ringbreaker, Template_relevance Reaxys, Template_relevance Reaxys_biocatalysis model, leveraging a vast database of chemical reactions to predict feasible synthetic routes.
One-Step Synthesis Focus: Specifically designed for one-step synthesis, it provides concise and direct routes for your target compounds, streamlining the synthesis process.
Accurate Predictions: Utilizing the extensive PISTACHIO, BKMS_METABOLIC, PISTACHIO_RINGBREAKER, REAXYS, REAXYS_BIOCATALYSIS database, our tool offers high-accuracy predictions, reflecting the latest in chemical research and data.
Strategy Settings
Precursor scoring | Relevance Heuristic |
---|---|
Min. plausibility | 0.01 |
Model | Template_relevance |
Template Set | Pistachio/Bkms_metabolic/Pistachio_ringbreaker/Reaxys/Reaxys_biocatalysis |
Top-N result to add to graph | 6 |
Feasible Synthetic Routes
Disclaimer and Information on In-Vitro Research Products
Please be aware that all articles and product information presented on BenchChem are intended solely for informational purposes. The products available for purchase on BenchChem are specifically designed for in-vitro studies, which are conducted outside of living organisms. In-vitro studies, derived from the Latin term "in glass," involve experiments performed in controlled laboratory settings using cells or tissues. It is important to note that these products are not categorized as medicines or drugs, and they have not received approval from the FDA for the prevention, treatment, or cure of any medical condition, ailment, or disease. We must emphasize that any form of bodily introduction of these products into humans or animals is strictly prohibited by law. It is essential to adhere to these guidelines to ensure compliance with legal and ethical standards in research and experimentation.