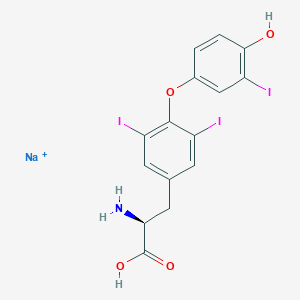
Liothyronine sodium
Overview
Description
Liothyronine sodium is a synthetic form of the thyroid hormone triiodothyronine (T3). It is commonly used in the treatment of hypothyroidism, a condition where the thyroid gland does not produce enough thyroid hormone. This compound is known for its rapid onset of action and is often used when a quick response is needed, such as in cases of myxedema coma .
Preparation Methods
Synthetic Routes and Reaction Conditions
Liothyronine sodium is synthesized through a multi-step chemical process. The synthesis typically involves the iodination of tyrosine derivatives. The key steps include:
Iodination: Tyrosine is iodinated to form monoiodotyrosine and diiodotyrosine.
Coupling: These iodinated intermediates are then coupled to form triiodothyronine.
Purification: The final product is purified through crystallization and other purification techniques to obtain this compound in its pure form.
Industrial Production Methods
In industrial settings, the production of this compound involves large-scale chemical reactors and stringent quality control measures. The process is optimized for high yield and purity, ensuring that the final product meets pharmaceutical standards. The industrial production also involves the use of advanced purification techniques such as high-performance liquid chromatography (HPLC) to ensure the removal of impurities .
Chemical Reactions Analysis
Absorption and Distribution
- Absorption : Liothyronine sodium is nearly completely absorbed (95%) from the gastrointestinal tract, with peak plasma concentrations (Cmax) achieved within 120 minutes .
- Protein Binding : Unlike levothyroxine (T4), liothyronine is not firmly bound to plasma proteins, enabling rapid tissue distribution .
Pharmacokinetic Parameters :
Parameter | Value (Mean ± SD) |
---|---|
Plasma Half-Life | 1–2 days |
T1/2 (IV) | 216 ± 51 minutes |
Volume of Distribution | 45 ± 13 mL/min |
Conversion in Peripheral Tissues
- Deiodination : T3 is inactivated via removal of iodine atoms by deiodinases, producing reverse T3 (rT3) and other metabolites .
- Conjugation : T3 undergoes glucuronidation and sulfation in the liver for excretion .
Reaction Example :
Stability and Degradation
- Storage Conditions : this compound tablets must be stored at 15–30°C to maintain stability. Injectable forms require refrigeration (2–8°C) .
- Hydrolysis : The sodium salt is susceptible to hydrolysis in acidic or basic conditions, leading to degradation .
Degradation Pathway :
Hormone-Receptor Interactions
Liothyronine binds to nuclear thyroid hormone receptors (TRα/β), activating transcription of genes involved in metabolism, growth, and differentiation .
Mechanism :
Adverse Reactions
Excess liothyronine triggers hyperthyroidism-like effects, including:
- Cardiovascular : Increased heart rate (tachycardia) and arrhythmias .
- Metabolic : Weight loss and hyperthermia .
Toxicity Threshold :
Key Research Findings
- Pharmacokinetic Variability : A study comparing liothyronine and levothyroxine found T3 levels peaked within 2 hours post-administration, with no significant differences in cardiovascular parameters .
- Therapeutic Efficacy : Liothyronine therapy reduced body weight by 2.3 kg over 12 weeks in hypothyroid patients compared to T4 monotherapy .
Scientific Research Applications
Treatment of Hypothyroidism
Liothyronine sodium is indicated for treating hypothyroidism, especially in patients who do not respond adequately to levothyroxine (LT4) alone. It can be used as a replacement therapy or in combination with LT4 to optimize thyroid hormone levels. Studies have shown that some patients experience improved symptoms when liothyronine is added to their treatment regimen, particularly those with persistent fatigue and cognitive dysfunction despite normal TSH levels .
Case Study: Combination Therapy
A clinical case demonstrated that a patient with primary hypothyroidism who was unresponsive to LT4 alone showed significant improvement in energy levels and mood after the addition of this compound to their treatment plan. This highlights the importance of personalized medicine in managing thyroid disorders.
Management of Myxedema Coma
This compound is critical in treating myxedema coma, a life-threatening condition resulting from severe hypothyroidism. The rapid action of T3 allows for quick restoration of metabolic functions in critically ill patients. In emergency settings, intravenous administration of liothyronine can lead to rapid clinical improvement .
Clinical Protocol
In cases of myxedema coma, the standard protocol involves administering liothyronine intravenously at doses ranging from 5 to 20 micrograms, depending on the patient's response and severity of the condition.
Thyrotoxicosis Management
This compound can serve as an adjunct therapy in managing thyrotoxicosis, particularly during treatment with antithyroid medications like carbimazole. It helps prevent subclinical hypothyroidism that may develop during the course of treatment .
Efficacy Evidence
Clinical trials have indicated that using liothyronine alongside carbimazole can effectively manage thyroid hormone levels without exacerbating symptoms of hyperthyroidism.
Research and Development in Novel Delivery Systems
Recent advancements have focused on improving the delivery mechanisms for this compound to enhance its therapeutic efficacy and minimize side effects. Various formulations are being explored, including:
- Sustained Release Formulations: These aim to maintain stable T3 levels over extended periods, reducing peaks and troughs associated with conventional dosing .
- Nanoparticle Encapsulation: Research has shown that encapsulating liothyronine in nanoparticles can enhance its delivery to specific tissues, such as the brain, potentially improving outcomes in neurodegenerative conditions .
Cardiovascular Applications
Emerging studies suggest that this compound may have beneficial effects on cardiovascular health, particularly in patients with heart failure and low thyroid hormone levels. T3 has been shown to improve cardiac contractility and reduce systemic vascular resistance .
Clinical Observations
Patients with heart failure receiving liothyronine therapy demonstrated improved exercise capacity and quality of life metrics compared to those on standard heart failure treatments alone.
Research Findings Summary Table
Application Area | Description | Key Findings |
---|---|---|
Hypothyroidism | Replacement or adjunct therapy for LT4 | Improved symptoms in resistant cases |
Myxedema Coma | Emergency treatment for severe hypothyroidism | Rapid clinical improvement with IV administration |
Thyrotoxicosis | Adjunct therapy during antithyroid treatment | Prevents subclinical hypothyroidism |
Novel Delivery Systems | Sustained release and nanoparticle formulations | Enhanced therapeutic efficacy and targeted delivery |
Cardiovascular Health | Potential benefits in heart failure management | Improved cardiac function and patient quality of life |
Mechanism of Action
Liothyronine sodium exerts its effects by mimicking the action of natural thyroid hormone triiodothyronine (T3). It binds to thyroid hormone receptors in the nucleus of cells, leading to the activation of specific genes involved in metabolism, growth, and development. The molecular targets include thyroid hormone receptors (TRα and TRβ), which regulate the transcription of genes involved in energy metabolism and protein synthesis .
Comparison with Similar Compounds
Similar Compounds
Levothyroxine Sodium: Another synthetic thyroid hormone, but it mimics thyroxine (T4) rather than triiodothyronine (T3).
Desiccated Thyroid Extract: A natural product derived from animal thyroid glands, containing both T3 and T4.
Uniqueness
Liothyronine sodium is unique in its rapid onset of action compared to levothyroxine sodium, making it particularly useful in emergency situations such as myxedema coma. It is also more potent on a per-microgram basis, providing a stronger and quicker therapeutic effect .
Biological Activity
Liothyronine sodium, a synthetic form of the thyroid hormone triiodothyronine (T3), is primarily used in the treatment of hypothyroidism and other thyroid-related disorders. Its biological activity is characterized by its rapid onset of action and profound effects on metabolism and cellular function. This article explores the mechanisms, pharmacokinetics, clinical applications, and case studies related to the biological activity of this compound.
This compound exerts its physiological effects by binding to thyroid hormone receptors located in the nucleus of target cells. This binding initiates a cascade of biological processes:
- Gene Transcription : Liothyronine regulates gene expression by activating thyroid hormone response elements on DNA, leading to increased transcription of genes involved in metabolism and growth .
- Protein Synthesis : The hormone enhances protein synthesis, influencing various metabolic pathways including carbohydrate and lipid metabolism .
- Cellular Metabolism : Liothyronine increases the basal metabolic rate (BMR) and oxygen consumption across tissues, significantly impacting energy expenditure and thermogenesis .
Pharmacokinetics
The pharmacokinetic profile of this compound is crucial for understanding its biological activity:
- Absorption : Approximately 95% of liothyronine is absorbed within four hours post-administration, allowing for rapid therapeutic effects .
- Distribution : Unlike thyroxine (T4), liothyronine is not firmly bound to serum proteins, making it more readily available to tissues .
- Half-life : The biological half-life of liothyronine is about 2.5 days, which facilitates quick dosage adjustments in clinical settings .
Clinical Applications
This compound is utilized in various clinical scenarios:
- Hypothyroidism Treatment : It serves as a replacement therapy for patients with insufficient endogenous thyroid hormone production.
- TSH Suppression : It is used to suppress thyroid-stimulating hormone (TSH) levels in certain thyroid cancers.
- Diagnostic Aid : Liothyronine can assist in diagnosing hyperthyroidism by evaluating the responsiveness of the pituitary gland to thyroid hormones .
Case Study 1: Liothyronine-Induced Thyrotoxicosis
A 29-year-old bodybuilder experienced symptoms consistent with thyrotoxicosis after long-term use of liothyronine at doses between 50-100 µg daily. Blood tests revealed significant hypokalemia, prompting potassium supplementation and cessation of liothyronine use. The patient recovered without further complications .
Case Study 2: Iatrogenic Thyrotoxicosis
Another case involved a patient who developed iatrogenic thyrotoxicosis due to compounded liothyronine capsules. The patient presented with hypernatremia and hypokalemia, requiring hospitalization for electrolyte management. This case highlighted the risks associated with compounded medications and the need for careful monitoring during treatment .
Case Study 3: Liothyronine Augmentation in Depression
A 17-year-old female was prescribed liothyronine as an adjunct therapy for depression but subsequently developed symptoms indicative of thyrotoxicosis. This case underscored the necessity for close monitoring when using liothyronine, especially in populations such as adolescents where atypical laboratory results may arise .
Summary of Research Findings
The following table summarizes key pharmacological data regarding this compound:
Parameter | Value |
---|---|
Absorption | ~95% within 4 hours |
Biological Half-life | ~2.5 days |
Maximum Pharmacologic Response | 2-3 days |
Mechanism | Agonist at thyroid receptors |
Primary Actions | Increases BMR, protein synthesis, cellular metabolism |
Q & A
Basic Research Questions
Q. What are the key chemical and pharmacological properties of liothyronine sodium relevant to experimental design?
this compound (C₁₅H₁₁I₃NNaO₄; MW 672.96) is the sodium salt of triiodothyronine (T₃), a potent thyroid hormone receptor (THR) agonist with higher affinity for THR-β1 than THR-α . Its stability in aqueous solutions is pH-dependent, requiring controlled storage conditions (e.g., pH 8–9, protected from light) to prevent deiodination . Researchers must account for its short half-life (~1 day in vitro) when designing time-course studies .
Methodological Guidance :
- Use high-performance liquid chromatography (HPLC) with UV detection (λ = 225 nm) for quantification .
- Validate purity thresholds (≤2.0% liothyronine impurity) per pharmacopeial standards using reference solutions .
Q. How does this compound’s mechanism of action influence receptor-binding assays?
this compound binds nuclear thyroid hormone receptors (THR-α/β), modulating gene transcription via thyroid response elements . Its lipophilic nature requires solvent systems (e.g., DMSO) to enhance cellular uptake in in vitro assays.
Methodological Guidance :
- Optimize cell permeability by pre-treating cultures with serum-free media to reduce protein binding interference .
- Include negative controls with reverse T₃ (rT₃) to distinguish receptor-specific effects .
Q. What analytical methods are recommended for assessing this compound stability in research formulations?
Stability studies should employ:
- HPLC-MS : To track degradation products (e.g., diiodothyronine) under stress conditions (heat, light, acidic pH) .
- Karl Fischer titration : For monitoring water content in lyophilized formulations, as hydration accelerates deiodination .
Advanced Research Questions
Q. How can researchers resolve contradictions in this compound’s dose-response data across studies?
Discrepancies often arise from:
- Receptor isoform variability : THR-β1 dominance in hepatic cells vs. THR-α in cardiac tissues .
- Species-specific metabolism : Rodents exhibit faster T₃ clearance than humans .
Methodological Guidance :
- Standardize models using THR-β1 knockout mice to isolate isoform-specific effects .
- Apply meta-analysis tools (e.g., RevMan) to harmonize dose-response data across species .
Q. What experimental design considerations are critical for in vivo vs. in vitro studies of this compound?
- In vivo : Monitor serum thyroid-stimulating hormone (TSH) suppression as a biomarker of systemic activity. Use pair-fed controls to distinguish metabolic effects from appetite changes .
- In vitro : Account for serum interference (e.g., thyroxine-binding globulin in cell media) by using charcoal-stripped fetal bovine serum .
Methodological Guidance :
- For chronic studies, employ osmotic minipumps to maintain stable plasma concentrations, avoiding peak-trough artifacts .
Q. How can researchers address challenges in quantifying this compound’s off-target effects?
Off-target effects (e.g., cardiac arrhythmias) may stem from THR-α activation. Strategies include:
- CRISPR-Cas9 silencing : To dissect receptor-specific pathways in cardiomyocytes .
- Transcriptomic profiling : RNA-seq to identify non-canonical signaling nodes (e.g., integrin αVβ3) .
Q. What statistical models are optimal for analyzing this compound’s non-linear pharmacokinetics?
Use non-compartmental analysis (NCA) for initial profiling, followed by physiologically based pharmacokinetic (PBPK) modeling to incorporate tissue-specific uptake and enterohepatic recirculation .
Methodological Guidance :
Q. How can this compound research be optimized for translational impact?
Properties
CAS No. |
55-06-1 |
---|---|
Molecular Formula |
C15H12I3NNaO4 |
Molecular Weight |
673.96 g/mol |
IUPAC Name |
sodium;(2S)-2-amino-3-[4-(4-hydroxy-3-iodophenoxy)-3,5-diiodophenyl]propanoate |
InChI |
InChI=1S/C15H12I3NO4.Na/c16-9-6-8(1-2-13(9)20)23-14-10(17)3-7(4-11(14)18)5-12(19)15(21)22;/h1-4,6,12,20H,5,19H2,(H,21,22);/t12-;/m0./s1 |
InChI Key |
LXHCVQFUTOUZEQ-YDALLXLXSA-N |
SMILES |
C1=CC(=C(C=C1OC2=C(C=C(C=C2I)CC(C(=O)O)N)I)I)O.[Na+] |
Isomeric SMILES |
C1=CC(=C(C=C1OC2=C(C=C(C=C2I)C[C@@H](C(=O)O)N)I)I)O.[Na] |
Canonical SMILES |
C1=CC(=C(C=C1OC2=C(C=C(C=C2I)CC(C(=O)O)N)I)I)O.[Na] |
Appearance |
Assay:≥98%A crystalline solid |
Key on ui other cas no. |
55-06-1 |
Pictograms |
Irritant; Health Hazard |
Synonyms |
3,3',5-Triiodothyronine Cytomel Liothyronine Liothyronine Sodium T3 Thyroid Hormone Thyroid Hormone, T3 Triiodothyronine |
Origin of Product |
United States |
Retrosynthesis Analysis
AI-Powered Synthesis Planning: Our tool employs the Template_relevance Pistachio, Template_relevance Bkms_metabolic, Template_relevance Pistachio_ringbreaker, Template_relevance Reaxys, Template_relevance Reaxys_biocatalysis model, leveraging a vast database of chemical reactions to predict feasible synthetic routes.
One-Step Synthesis Focus: Specifically designed for one-step synthesis, it provides concise and direct routes for your target compounds, streamlining the synthesis process.
Accurate Predictions: Utilizing the extensive PISTACHIO, BKMS_METABOLIC, PISTACHIO_RINGBREAKER, REAXYS, REAXYS_BIOCATALYSIS database, our tool offers high-accuracy predictions, reflecting the latest in chemical research and data.
Strategy Settings
Precursor scoring | Relevance Heuristic |
---|---|
Min. plausibility | 0.01 |
Model | Template_relevance |
Template Set | Pistachio/Bkms_metabolic/Pistachio_ringbreaker/Reaxys/Reaxys_biocatalysis |
Top-N result to add to graph | 6 |
Feasible Synthetic Routes
Disclaimer and Information on In-Vitro Research Products
Please be aware that all articles and product information presented on BenchChem are intended solely for informational purposes. The products available for purchase on BenchChem are specifically designed for in-vitro studies, which are conducted outside of living organisms. In-vitro studies, derived from the Latin term "in glass," involve experiments performed in controlled laboratory settings using cells or tissues. It is important to note that these products are not categorized as medicines or drugs, and they have not received approval from the FDA for the prevention, treatment, or cure of any medical condition, ailment, or disease. We must emphasize that any form of bodily introduction of these products into humans or animals is strictly prohibited by law. It is essential to adhere to these guidelines to ensure compliance with legal and ethical standards in research and experimentation.