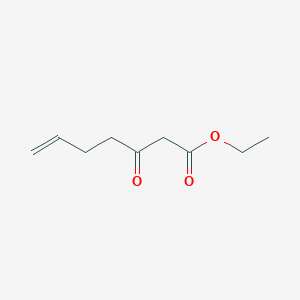
ethyl 3-oxohept-6-enoate
Overview
Description
ethyl 3-oxohept-6-enoate is an organic compound with the molecular formula C9H14O3. It is a colorless liquid that is used in various chemical reactions and research applications. The compound is characterized by the presence of an ester group, a ketone group, and a double bond in its structure.
Mechanism of Action
Ethyl 3-oxohept-6-enoate, also known as Ethyl 3-oxo-6-heptenoate, is a chemical compound with the molecular formula C9H14O3 . This article will discuss its mechanism of action, including its targets, mode of action, biochemical pathways, pharmacokinetics, results of action, and the influence of environmental factors.
Pharmacokinetics
Some physicochemical properties of the compound have been reported . It has a high gastrointestinal absorption and is predicted to be BBB (Blood-Brain Barrier) permeant . Its lipophilicity (Log Po/w) is 2.29 (iLOGP), 1.67 (XLOGP3), 1.47 (WLOGP), 1.19 (MLOGP), and 1.96 (SILICOS-IT), with a consensus Log Po/w of 1.72 . These properties may impact the compound’s bioavailability.
Action Environment
It is known that the compound should be stored at a temperature of +4°c .
Preparation Methods
Synthetic Routes and Reaction Conditions
ethyl 3-oxohept-6-enoate can be synthesized through several methods. One common method involves the reaction of ethyl acetoacetate with allyl bromide in the presence of a base such as sodium ethoxide. The reaction proceeds through a nucleophilic substitution mechanism, resulting in the formation of ethyl 3-oxo-6-heptenoate.
Another method involves the use of zinc-copper couple and benzene as solvents. Ethyl 4-iodobutyrate is reacted with methacryloyl chloride in the presence of tetrakis(triphenylphosphine)palladium(0) as a catalyst. The reaction is carried out under reflux conditions, and the product is purified through chromatography and distillation .
Industrial Production Methods
Industrial production of ethyl 3-oxo-6-heptenoate typically involves large-scale synthesis using similar methods as described above. The reaction conditions are optimized to ensure high yield and purity of the product. The use of continuous flow reactors and automated systems can enhance the efficiency and scalability of the production process.
Chemical Reactions Analysis
Types of Reactions
ethyl 3-oxohept-6-enoate undergoes various chemical reactions, including:
Oxidation: The compound can be oxidized to form corresponding carboxylic acids or other oxidized derivatives.
Reduction: Reduction reactions can convert the ketone group to an alcohol group, resulting in the formation of ethyl 3-hydroxy-6-heptenoate.
Substitution: The ester group can undergo nucleophilic substitution reactions, leading to the formation of different derivatives.
Common Reagents and Conditions
Oxidation: Common oxidizing agents include potassium permanganate and chromium trioxide.
Reduction: Reducing agents such as sodium borohydride and lithium aluminum hydride are commonly used.
Substitution: Nucleophiles such as amines and alcohols can be used in substitution reactions.
Major Products Formed
Oxidation: Carboxylic acids and other oxidized derivatives.
Reduction: Ethyl 3-hydroxy-6-heptenoate.
Substitution: Various ester and amide derivatives.
Scientific Research Applications
ethyl 3-oxohept-6-enoate has several scientific research applications, including:
Chemistry: It is used as an intermediate in the synthesis of various organic compounds. Its reactivity makes it a valuable building block in organic synthesis.
Biology: The compound can be used in the study of enzyme-catalyzed reactions and metabolic pathways.
Industry: this compound is used in the production of fine chemicals, fragrances, and flavoring agents.
Comparison with Similar Compounds
ethyl 3-oxohept-6-enoate can be compared with similar compounds such as:
Ethyl acetoacetate: Both compounds contain ester and ketone groups, but ethyl 3-oxo-6-heptenoate has an additional double bond.
Methyl 3-oxo-6-heptenoate: Similar structure but with a methyl ester group instead of an ethyl ester group.
Ethyl 3-oxo-4-pentenoate: Similar structure but with a shorter carbon chain.
The uniqueness of ethyl 3-oxo-6-heptenoate lies in its specific combination of functional groups and its reactivity, making it a versatile compound in various chemical reactions and applications.
Properties
IUPAC Name |
ethyl 3-oxohept-6-enoate | |
---|---|---|
Source | PubChem | |
URL | https://pubchem.ncbi.nlm.nih.gov | |
Description | Data deposited in or computed by PubChem | |
InChI |
InChI=1S/C9H14O3/c1-3-5-6-8(10)7-9(11)12-4-2/h3H,1,4-7H2,2H3 | |
Source | PubChem | |
URL | https://pubchem.ncbi.nlm.nih.gov | |
Description | Data deposited in or computed by PubChem | |
InChI Key |
BKXPKNYXXNYCBX-UHFFFAOYSA-N | |
Source | PubChem | |
URL | https://pubchem.ncbi.nlm.nih.gov | |
Description | Data deposited in or computed by PubChem | |
Canonical SMILES |
CCOC(=O)CC(=O)CCC=C | |
Source | PubChem | |
URL | https://pubchem.ncbi.nlm.nih.gov | |
Description | Data deposited in or computed by PubChem | |
Molecular Formula |
C9H14O3 | |
Source | PubChem | |
URL | https://pubchem.ncbi.nlm.nih.gov | |
Description | Data deposited in or computed by PubChem | |
DSSTOX Substance ID |
DTXSID40289670 | |
Record name | Ethyl 3-oxo-6-heptenoate | |
Source | EPA DSSTox | |
URL | https://comptox.epa.gov/dashboard/DTXSID40289670 | |
Description | DSSTox provides a high quality public chemistry resource for supporting improved predictive toxicology. | |
Molecular Weight |
170.21 g/mol | |
Source | PubChem | |
URL | https://pubchem.ncbi.nlm.nih.gov | |
Description | Data deposited in or computed by PubChem | |
CAS No. |
17605-06-0 | |
Record name | Ethyl 3-oxo-6-heptenoate | |
Source | DTP/NCI | |
URL | https://dtp.cancer.gov/dtpstandard/servlet/dwindex?searchtype=NSC&outputformat=html&searchlist=62677 | |
Description | The NCI Development Therapeutics Program (DTP) provides services and resources to the academic and private-sector research communities worldwide to facilitate the discovery and development of new cancer therapeutic agents. | |
Explanation | Unless otherwise indicated, all text within NCI products is free of copyright and may be reused without our permission. Credit the National Cancer Institute as the source. | |
Record name | Ethyl 3-oxo-6-heptenoate | |
Source | EPA DSSTox | |
URL | https://comptox.epa.gov/dashboard/DTXSID40289670 | |
Description | DSSTox provides a high quality public chemistry resource for supporting improved predictive toxicology. | |
Record name | Ethyl 3-oxo-6-heptenoate | |
Source | European Chemicals Agency (ECHA) | |
URL | https://echa.europa.eu/information-on-chemicals | |
Description | The European Chemicals Agency (ECHA) is an agency of the European Union which is the driving force among regulatory authorities in implementing the EU's groundbreaking chemicals legislation for the benefit of human health and the environment as well as for innovation and competitiveness. | |
Explanation | Use of the information, documents and data from the ECHA website is subject to the terms and conditions of this Legal Notice, and subject to other binding limitations provided for under applicable law, the information, documents and data made available on the ECHA website may be reproduced, distributed and/or used, totally or in part, for non-commercial purposes provided that ECHA is acknowledged as the source: "Source: European Chemicals Agency, http://echa.europa.eu/". Such acknowledgement must be included in each copy of the material. ECHA permits and encourages organisations and individuals to create links to the ECHA website under the following cumulative conditions: Links can only be made to webpages that provide a link to the Legal Notice page. | |
Retrosynthesis Analysis
AI-Powered Synthesis Planning: Our tool employs the Template_relevance Pistachio, Template_relevance Bkms_metabolic, Template_relevance Pistachio_ringbreaker, Template_relevance Reaxys, Template_relevance Reaxys_biocatalysis model, leveraging a vast database of chemical reactions to predict feasible synthetic routes.
One-Step Synthesis Focus: Specifically designed for one-step synthesis, it provides concise and direct routes for your target compounds, streamlining the synthesis process.
Accurate Predictions: Utilizing the extensive PISTACHIO, BKMS_METABOLIC, PISTACHIO_RINGBREAKER, REAXYS, REAXYS_BIOCATALYSIS database, our tool offers high-accuracy predictions, reflecting the latest in chemical research and data.
Strategy Settings
Precursor scoring | Relevance Heuristic |
---|---|
Min. plausibility | 0.01 |
Model | Template_relevance |
Template Set | Pistachio/Bkms_metabolic/Pistachio_ringbreaker/Reaxys/Reaxys_biocatalysis |
Top-N result to add to graph | 6 |
Feasible Synthetic Routes
Q1: How does ethyl 3-oxo-6-heptenoate influence the stereoselectivity of β-keto ester reduction by baker's yeast?
A1: The research demonstrates that ethyl 3-oxo-6-heptenoate acts as an additive that significantly impacts the stereochemical outcome of β-keto ester reduction by baker's yeast []. Specifically, it promotes the formation of the D-hydroxy ester. While the exact mechanism remains unclear, the study suggests that ethyl 3-oxo-6-heptenoate, along with other α,β-unsaturated carbonyl compounds, might inhibit enzymes responsible for producing the L-hydroxy ester []. This inhibition shifts the reaction equilibrium towards the production of the D-isomer, resulting in high stereoselectivity. Further research is needed to confirm this hypothesis and elucidate the specific interactions between the compound and the involved enzymes.
Disclaimer and Information on In-Vitro Research Products
Please be aware that all articles and product information presented on BenchChem are intended solely for informational purposes. The products available for purchase on BenchChem are specifically designed for in-vitro studies, which are conducted outside of living organisms. In-vitro studies, derived from the Latin term "in glass," involve experiments performed in controlled laboratory settings using cells or tissues. It is important to note that these products are not categorized as medicines or drugs, and they have not received approval from the FDA for the prevention, treatment, or cure of any medical condition, ailment, or disease. We must emphasize that any form of bodily introduction of these products into humans or animals is strictly prohibited by law. It is essential to adhere to these guidelines to ensure compliance with legal and ethical standards in research and experimentation.