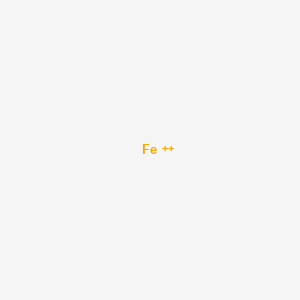
Ferrous ion
Overview
Description
Iron(2+) is a divalent metal cation, an iron cation and a monoatomic dication. It has a role as a human metabolite, a Saccharomyces cerevisiae metabolite, a mouse metabolite and a cofactor.
Fe2+ is a metabolite found in or produced by Escherichia coli (strain K12, MG1655).
Ferrous ion is a natural product found in Homo sapiens with data available.
Iron(2+) is a metabolite found in or produced by Saccharomyces cerevisiae.
See also: Ferrous Sulfate (active moiety of); Ferrous Fumarate (active moiety of); Iron pentacarbonyl (active moiety of) ... View More ...
Properties
CAS No. |
15438-31-0 |
---|---|
Molecular Formula |
Fe+2 |
Molecular Weight |
55.84 g/mol |
IUPAC Name |
iron(2+) |
InChI |
InChI=1S/Fe/q+2 |
InChI Key |
CWYNVVGOOAEACU-UHFFFAOYSA-N |
SMILES |
[Fe+2] |
Canonical SMILES |
[Fe+2] |
melting_point |
1538 °C |
15438-31-0 1317-63-1 68187-35-9 |
|
physical_description |
Other Solid Reddish-brown odorless powder; Insoluble in water; [Hoover Color MSDS] Solid |
Origin of Product |
United States |
Synthesis routes and methods I
Procedure details
Synthesis routes and methods II
Procedure details
Disclaimer and Information on In-Vitro Research Products
Please be aware that all articles and product information presented on BenchChem are intended solely for informational purposes. The products available for purchase on BenchChem are specifically designed for in-vitro studies, which are conducted outside of living organisms. In-vitro studies, derived from the Latin term "in glass," involve experiments performed in controlled laboratory settings using cells or tissues. It is important to note that these products are not categorized as medicines or drugs, and they have not received approval from the FDA for the prevention, treatment, or cure of any medical condition, ailment, or disease. We must emphasize that any form of bodily introduction of these products into humans or animals is strictly prohibited by law. It is essential to adhere to these guidelines to ensure compliance with legal and ethical standards in research and experimentation.