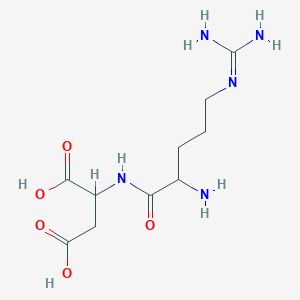
Arg-Asp
Overview
Description
The compound “Arg-Asp” refers to a dipeptide composed of the amino acids arginine and aspartic acid. This dipeptide is part of a larger family of peptides that play crucial roles in various biological processes. The this compound sequence is particularly significant in the context of cell adhesion, signaling, and interactions with integrins, which are receptors that mediate cell-extracellular matrix interactions.
Mechanism of Action
Target of Action
L-arginyl-L-aspartic acid, also known as H-ARG-ASP-OH or Arg-Asp, is a dipeptide composed of the amino acids arginine and aspartic acidIt’s known that peptides containing the arg-gly-asp (rgd) sequence are recognized by integrins, a family of cell adhesion receptors . Although H-ARG-ASP-OH lacks the Glycine (Gly) residue, it’s plausible that it may interact with similar targets due to the presence of Arginine (Arg) and Aspartic acid (Asp).
Mode of Action
Peptides containing the rgd sequence are known to interact with their targets (integrins) by binding to them, which results in changes in cell adhesion
Biochemical Pathways
For instance, arginine is a precursor for the synthesis of nitric oxide, a key signaling molecule, and aspartic acid is involved in the citric acid cycle .
Pharmacokinetics
The pharmacokinetics of H-ARG-ASP-OH, including its absorption, distribution, metabolism, and excretion (ADME) properties, are not well-studied. As a peptide, it’s likely to be absorbed in the gut and distributed throughout the body. It may be metabolized by peptidases and other enzymes, and excreted via the kidneys . .
Action Environment
Environmental factors can influence the action, efficacy, and stability of H-ARG-ASP-OH. For instance, factors such as pH and temperature can affect the stability of peptides. Additionally, the presence of other molecules, such as enzymes that can degrade peptides, can influence the action and efficacy of H-ARG-ASP-OH .
Biochemical Analysis
Biochemical Properties
L-Arginyl-L-Aspartic acid interacts with several enzymes, proteins, and other biomolecules. The Arg-Gly-Asp (RGD) cell adhesion sequence, which includes arginine and aspartic acid, is found in several extracellular matrix molecules known to interact with integrin cell-surface receptors . These interactions are key specificity determinants for integrin recognition, involving hydrogen bonding and metal coordination interactions .
Cellular Effects
The effects of L-Arginyl-L-Aspartic acid on various types of cells and cellular processes are profound. For instance, the RGD sequence, which includes arginine and aspartic acid, is recognized by members of the integrin family of cell surface receptors . Through these cell-matrix and cell-cell interactions, integrins control diverse cell functions such as adhesion, shape, growth, differentiation, and mobility .
Molecular Mechanism
L-Arginyl-L-Aspartic acid exerts its effects at the molecular level through various mechanisms. For instance, the interaction between the central Gly residue in the RGD sequence and a carbonyl group in the integrin surface shows all the hallmarks of Cα-H···O=C hydrogen bonding . This interaction contributes to the specificity of the RGD-integrin interaction and highlights the importance of the conserved Gly residue in the RGD motif .
Metabolic Pathways
L-Arginyl-L-Aspartic acid is involved in several metabolic pathways. For instance, the RGD sequence, which includes arginine and aspartic acid, is part of several extracellular matrix molecules . The metabolic pathways of these molecules could potentially involve L-Arginyl-L-Aspartic acid.
Subcellular Localization
Given its involvement in the RGD sequence and its interaction with integrin cell-surface receptors, it is plausible that L-Arginyl-L-Aspartic acid may be localized to areas where these interactions occur .
Preparation Methods
Synthetic Routes and Reaction Conditions
The synthesis of the Arg-Asp dipeptide typically involves solid-phase peptide synthesis (SPPS). This method allows for the sequential addition of amino acids to a growing peptide chain anchored to a solid resin. The process involves the following steps:
Coupling: The carboxyl group of aspartic acid is activated using a coupling reagent such as dicyclohexylcarbodiimide (DCC) or N,N’-diisopropylcarbodiimide (DIC) and then reacted with the amino group of arginine.
Deprotection: Protecting groups on the amino acids are removed to expose reactive sites for further coupling.
Cleavage: The completed peptide is cleaved from the resin using a cleavage reagent like trifluoroacetic acid (TFA).
Industrial Production Methods
In an industrial setting, the production of this compound dipeptide can be scaled up using automated peptide synthesizers. These machines automate the SPPS process, allowing for the efficient and reproducible synthesis of peptides in large quantities. The use of high-performance liquid chromatography (HPLC) ensures the purity of the final product.
Chemical Reactions Analysis
Types of Reactions
The Arg-Asp dipeptide can undergo various chemical reactions, including:
Oxidation: The guanidinium group of arginine can be oxidized to form nitric oxide, a signaling molecule.
Reduction: Reduction reactions can modify the carboxyl group of aspartic acid to form aspartate.
Substitution: The amino groups can participate in substitution reactions to form derivatives with different functional groups.
Common Reagents and Conditions
Oxidation: Hydrogen peroxide or peracids can be used as oxidizing agents.
Reduction: Sodium borohydride or lithium aluminum hydride can be used as reducing agents.
Substitution: Various alkylating agents can be used for substitution reactions.
Major Products Formed
Oxidation: Nitric oxide and other nitrogen oxides.
Reduction: Aspartate and reduced forms of arginine.
Substitution: Derivatives with modified functional groups.
Scientific Research Applications
Chemistry
In chemistry, the Arg-Asp dipeptide is used as a model compound to study peptide synthesis, structure, and reactivity. It serves as a building block for the synthesis of more complex peptides and proteins.
Biology
In biology, the this compound sequence is crucial for cell adhesion and signaling. It interacts with integrins, which are receptors that mediate cell-extracellular matrix interactions. This interaction is essential for processes such as wound healing, immune response, and tissue development.
Medicine
In medicine, the this compound dipeptide is used in the development of therapeutic agents that target integrin receptors. These agents can inhibit cell adhesion and migration, making them potential treatments for cancer and inflammatory diseases.
Industry
In the pharmaceutical industry, the this compound dipeptide is used in drug delivery systems. It can be conjugated to nanoparticles to enhance the targeting and delivery of drugs to specific cells or tissues.
Comparison with Similar Compounds
Similar Compounds
Arg-Gly-Asp: A tripeptide that includes glycine and has similar integrin-binding properties.
Cyclo(Arg-Gly-Asp-D-Phe-Val): A cyclic peptide with enhanced stability and binding affinity for integrins.
Arg-Gly-Asp-Ser: A tetrapeptide with additional serine residue that enhances its biological activity.
Uniqueness
The Arg-Asp dipeptide is unique in its simplicity and specificity for certain integrin receptors. While longer peptides like Arg-Gly-Asp and its derivatives have broader applications, this compound provides a more focused interaction with specific integrins, making it a valuable tool in targeted therapeutic applications.
Properties
IUPAC Name |
2-[[2-amino-5-(diaminomethylideneamino)pentanoyl]amino]butanedioic acid | |
---|---|---|
Details | Computed by LexiChem 2.6.6 (PubChem release 2019.06.18) | |
Source | PubChem | |
URL | https://pubchem.ncbi.nlm.nih.gov | |
Description | Data deposited in or computed by PubChem | |
InChI |
InChI=1S/C10H19N5O5/c11-5(2-1-3-14-10(12)13)8(18)15-6(9(19)20)4-7(16)17/h5-6H,1-4,11H2,(H,15,18)(H,16,17)(H,19,20)(H4,12,13,14) | |
Details | Computed by InChI 1.0.5 (PubChem release 2019.06.18) | |
Source | PubChem | |
URL | https://pubchem.ncbi.nlm.nih.gov | |
Description | Data deposited in or computed by PubChem | |
InChI Key |
SIFXMYAHXJGAFC-UHFFFAOYSA-N | |
Details | Computed by InChI 1.0.5 (PubChem release 2019.06.18) | |
Source | PubChem | |
URL | https://pubchem.ncbi.nlm.nih.gov | |
Description | Data deposited in or computed by PubChem | |
Canonical SMILES |
C(CC(C(=O)NC(CC(=O)O)C(=O)O)N)CN=C(N)N | |
Details | Computed by OEChem 2.1.5 (PubChem release 2019.06.18) | |
Source | PubChem | |
URL | https://pubchem.ncbi.nlm.nih.gov | |
Description | Data deposited in or computed by PubChem | |
Molecular Formula |
C10H19N5O5 | |
Details | Computed by PubChem 2.1 (PubChem release 2019.06.18) | |
Source | PubChem | |
URL | https://pubchem.ncbi.nlm.nih.gov | |
Description | Data deposited in or computed by PubChem | |
DSSTOX Substance ID |
DTXSID10431265 | |
Record name | Arg-Asp | |
Source | EPA DSSTox | |
URL | https://comptox.epa.gov/dashboard/DTXSID10431265 | |
Description | DSSTox provides a high quality public chemistry resource for supporting improved predictive toxicology. | |
Molecular Weight |
289.29 g/mol | |
Details | Computed by PubChem 2.1 (PubChem release 2021.05.07) | |
Source | PubChem | |
URL | https://pubchem.ncbi.nlm.nih.gov | |
Description | Data deposited in or computed by PubChem | |
CAS No. |
15706-88-4 | |
Record name | Arg-Asp | |
Source | EPA DSSTox | |
URL | https://comptox.epa.gov/dashboard/DTXSID10431265 | |
Description | DSSTox provides a high quality public chemistry resource for supporting improved predictive toxicology. | |
Retrosynthesis Analysis
AI-Powered Synthesis Planning: Our tool employs the Template_relevance Pistachio, Template_relevance Bkms_metabolic, Template_relevance Pistachio_ringbreaker, Template_relevance Reaxys, Template_relevance Reaxys_biocatalysis model, leveraging a vast database of chemical reactions to predict feasible synthetic routes.
One-Step Synthesis Focus: Specifically designed for one-step synthesis, it provides concise and direct routes for your target compounds, streamlining the synthesis process.
Accurate Predictions: Utilizing the extensive PISTACHIO, BKMS_METABOLIC, PISTACHIO_RINGBREAKER, REAXYS, REAXYS_BIOCATALYSIS database, our tool offers high-accuracy predictions, reflecting the latest in chemical research and data.
Strategy Settings
Precursor scoring | Relevance Heuristic |
---|---|
Min. plausibility | 0.01 |
Model | Template_relevance |
Template Set | Pistachio/Bkms_metabolic/Pistachio_ringbreaker/Reaxys/Reaxys_biocatalysis |
Top-N result to add to graph | 6 |
Feasible Synthetic Routes
Q1: How does the Arg-Asp motif contribute to the function of certain proteins?
A1: The this compound sequence is often found within functional domains of proteins, influencing their activity and interactions. For instance, in the enzyme levansucrase from Acetobacter diazotrophicus, Asp-309 within the conserved this compound-Pro (RDP) motif plays a crucial role in catalysis. Mutating this aspartic acid to asparagine significantly reduces the enzyme's catalytic efficiency, highlighting the importance of this residue for sucrose hydrolysis.
Q2: Can you provide an example of this compound involvement in protein-protein interactions?
A2: Research on the Staphylococcus aureus enzyme Sortase A (SrtA) provides insights into the role of this compound containing peptides in protein interactions. The pentapeptide Leu-Pro-Arg-Asp-Ala (LPRDA) exhibits antivirulence activity against S. aureus by interacting with SrtA. Molecular modeling studies suggest that LPRDA binds to a flexible region within SrtA, influencing the enzyme's dynamics and potentially interfering with its function.
Q3: What is the significance of this compound pairings in protein structures?
A3: Analysis of protein structures reveals that this compound pairings, stabilized by salt bridges, are prevalent, often found within Arg-Arg-Asp/Glu clusters. These clusters frequently occur in polar environments, indicating a role for electrostatic interactions and solvation in their stability. The presence of acidic residues near Arg-Arg pairings further enhances their stability.
Q4: What is the molecular weight of the this compound dipeptide?
A4: The molecular weight of the this compound dipeptide is approximately 290.3 g/mol.
Q5: Are there any enzymes known to specifically cleave at the this compound bond?
A5: Yes, certain enzymes exhibit specificity for cleaving peptide bonds involving arginine and aspartic acid. For example, a dipeptidase isolated from human urine, identified as the released form of renal dipeptidase, efficiently cleaves the this compound bond. This enzyme, composed of four identical subunits, plays a role in the metabolism of dipeptides containing this specific amino acid sequence.
Q6: How have computational methods been used to study this compound containing peptides?
A6: Computational techniques, such as simulated annealing and molecular dynamics simulations, have been instrumental in studying the conformational preferences of this compound containing peptides. For example, researchers investigated the conformational space of the antithrombotic peptide Lys-Arg-Asp-Ser (KRDS) and its analogs using simulated annealing. They identified predominant conformational classes and related them to structural features determined from NMR spectroscopy. This study provided insights into the structure-activity relationships of these peptides.
Q7: How does altering the amino acid sequence around this compound affect biological activity?
A7: Modifications to the amino acid sequence surrounding this compound can significantly impact biological activity. In the context of antithrombotic peptides, replacing L-lysine with D-lysine in KRDS (yielding KDRDS) abolishes its activity, highlighting the importance of stereochemistry. Conversely, substituting aspartic acid and serine with glutamic acid in KRDS (resulting in KREE) maintains and even enhances the peptide's antithrombotic properties. These findings underscore the crucial role of neighboring residues in determining peptide activity and selectivity.
Disclaimer and Information on In-Vitro Research Products
Please be aware that all articles and product information presented on BenchChem are intended solely for informational purposes. The products available for purchase on BenchChem are specifically designed for in-vitro studies, which are conducted outside of living organisms. In-vitro studies, derived from the Latin term "in glass," involve experiments performed in controlled laboratory settings using cells or tissues. It is important to note that these products are not categorized as medicines or drugs, and they have not received approval from the FDA for the prevention, treatment, or cure of any medical condition, ailment, or disease. We must emphasize that any form of bodily introduction of these products into humans or animals is strictly prohibited by law. It is essential to adhere to these guidelines to ensure compliance with legal and ethical standards in research and experimentation.