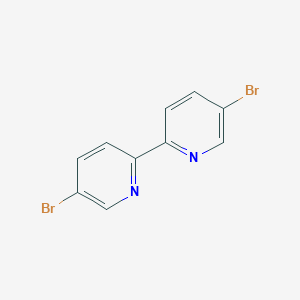
5,5'-Dibromo-2,2'-bipyridine
Overview
Description
Dichlorofluorescein is an organic dye belonging to the fluorescein family, characterized by the substitution of chlorine atoms at the 2 and 7 positions. It is widely used as an indicator in various analytical methods, particularly in argentometry by the Fajans method. The compound is known for its ability to shift color from colorless to faint pink upon reaching the equivalence point in titration reactions .
Mechanism of Action
Target of Action
The primary targets of 5,5’-Dibromo-2,2’-bipyridine are metal ions, particularly transition metals . The compound has excellent coordination properties, making it a versatile building block for the synthesis of functional materials .
Mode of Action
5,5’-Dibromo-2,2’-bipyridine interacts with its targets through metal-catalyzed coupling reactions . This interaction allows the compound to form larger conjugated molecules, which can be further functionalized .
Biochemical Pathways
The compound’s interaction with metal ions can affect various biochemical pathways. For instance, bipyridine derivatives can react with borane species to form organoboron compounds, which act as electron acceptors in organic photovoltaics .
Result of Action
The molecular and cellular effects of 5,5’-Dibromo-2,2’-bipyridine’s action are largely dependent on its targets and the specific biochemical pathways it affects. For example, when used in the synthesis of functional materials, the compound can contribute to the photoluminescence and electrochemiluminescence properties of these materials .
Action Environment
Environmental factors can influence the action, efficacy, and stability of 5,5’-Dibromo-2,2’-bipyridine. For instance, the compound should be stored in a dry environment at room temperature for optimal stability . Furthermore, the efficiency of the metal-catalyzed coupling reactions that the compound undergoes can be influenced by factors such as temperature and pressure .
Biochemical Analysis
Biochemical Properties
5,5’-Dibromo-2,2’-bipyridine has excellent coordination properties, making it a versatile building block for the synthesis of functional materials .
Cellular Effects
The effects of 5,5’-Dibromo-2,2’-bipyridine on cells and cellular processes are complex and multifaceted. It can influence cell function in various ways, potentially impacting cell signaling pathways, gene expression, and cellular metabolism .
Molecular Mechanism
At the molecular level, 5,5’-Dibromo-2,2’-bipyridine exerts its effects through a variety of mechanisms. These may include binding interactions with biomolecules, enzyme inhibition or activation, and changes in gene expression .
Temporal Effects in Laboratory Settings
In laboratory settings, the effects of 5,5’-Dibromo-2,2’-bipyridine can change over time. This may include changes in the product’s stability, degradation, and long-term effects on cellular function observed in in vitro or in vivo studies .
Dosage Effects in Animal Models
The effects of 5,5’-Dibromo-2,2’-bipyridine can vary with different dosages in animal models . This could include any threshold effects observed in these studies, as well as any toxic or adverse effects at high doses .
Metabolic Pathways
5,5’-Dibromo-2,2’-bipyridine may be involved in various metabolic pathways, interacting with enzymes or cofactors . It could also have effects on metabolic flux or metabolite levels .
Transport and Distribution
5,5’-Dibromo-2,2’-bipyridine can be transported and distributed within cells and tissues . This could involve interaction with transporters or binding proteins, and could affect its localization or accumulation .
Subcellular Localization
The subcellular localization of 5,5’-Dibromo-2,2’-bipyridine and its effects on activity or function are complex and multifaceted . This could involve targeting signals or post-translational modifications that direct it to specific compartments or organelles .
Preparation Methods
Synthetic Routes and Reaction Conditions: Dichlorofluorescein is synthesized through the reaction of fluorescein with chlorine gas. The process involves the chlorination of fluorescein in the presence of a suitable solvent, such as acetic acid, under controlled temperature conditions. The reaction typically proceeds as follows: [ \text{Fluorescein} + \text{Chlorine} \rightarrow \text{Dichlorofluorescein} ]
Industrial Production Methods: In industrial settings, the production of dichlorofluorescein involves large-scale chlorination reactors where fluorescein is exposed to chlorine gas under optimized conditions to ensure high yield and purity. The reaction is monitored and controlled to prevent over-chlorination and to achieve the desired product quality .
Chemical Reactions Analysis
Types of Reactions: Dichlorofluorescein undergoes various chemical reactions, including:
Oxidation: Dichlorofluorescein can be oxidized to form dichlorofluorescein diacetate, a fluorescent compound used in cellular assays.
Reduction: The compound can be reduced to its non-fluorescent form, dichlorofluorescin, which is used as a probe for detecting reactive oxygen species.
Substitution: Dichlorofluorescein can participate in substitution reactions where the chlorine atoms are replaced by other functional groups.
Common Reagents and Conditions:
Oxidation: Hydrogen peroxide (H₂O₂) in the presence of a peroxidase enzyme.
Reduction: Reducing agents such as sodium borohydride (NaBH₄).
Substitution: Nucleophiles such as amines or thiols under basic conditions.
Major Products Formed:
Oxidation: Dichlorofluorescein diacetate.
Reduction: Dichlorofluorescin.
Substitution: Various substituted fluorescein derivatives
Scientific Research Applications
Dichlorofluorescein has a wide range of applications in scientific research, including:
Chemistry: Used as an indicator in titration methods and as a fluorescent probe in various chemical assays.
Biology: Employed in cellular assays to measure reactive oxygen species and oxidative stress.
Medicine: Utilized in diagnostic assays and imaging techniques to detect cellular changes and disease markers.
Industry: Applied in the manufacturing of dye-sensitized solar cells and as a component in fluorescent dyes for various industrial applications
Comparison with Similar Compounds
Fluorescein: The parent compound of dichlorofluorescein, used in similar applications but lacks the chlorine substitutions.
Carboxyfluorescein: A derivative with carboxyl groups, used for pH detection in more acidic environments.
Dichlorofluorescein diacetate: A derivative used in cellular assays for detecting reactive oxygen species.
Uniqueness: Dichlorofluorescein is unique due to its chlorine substitutions, which enhance its fluorescent properties and make it more suitable for specific analytical and biological applications compared to its parent compound, fluorescein .
Properties
IUPAC Name |
5-bromo-2-(5-bromopyridin-2-yl)pyridine | |
---|---|---|
Source | PubChem | |
URL | https://pubchem.ncbi.nlm.nih.gov | |
Description | Data deposited in or computed by PubChem | |
InChI |
InChI=1S/C10H6Br2N2/c11-7-1-3-9(13-5-7)10-4-2-8(12)6-14-10/h1-6H | |
Source | PubChem | |
URL | https://pubchem.ncbi.nlm.nih.gov | |
Description | Data deposited in or computed by PubChem | |
InChI Key |
JNWPRPLNUUMYCM-UHFFFAOYSA-N | |
Source | PubChem | |
URL | https://pubchem.ncbi.nlm.nih.gov | |
Description | Data deposited in or computed by PubChem | |
Canonical SMILES |
C1=CC(=NC=C1Br)C2=NC=C(C=C2)Br | |
Source | PubChem | |
URL | https://pubchem.ncbi.nlm.nih.gov | |
Description | Data deposited in or computed by PubChem | |
Molecular Formula |
C10H6Br2N2 | |
Source | PubChem | |
URL | https://pubchem.ncbi.nlm.nih.gov | |
Description | Data deposited in or computed by PubChem | |
DSSTOX Substance ID |
DTXSID90447824 | |
Record name | 5,5'-DIBROMO-2,2'-BIPYRIDINE | |
Source | EPA DSSTox | |
URL | https://comptox.epa.gov/dashboard/DTXSID90447824 | |
Description | DSSTox provides a high quality public chemistry resource for supporting improved predictive toxicology. | |
Molecular Weight |
313.98 g/mol | |
Source | PubChem | |
URL | https://pubchem.ncbi.nlm.nih.gov | |
Description | Data deposited in or computed by PubChem | |
CAS No. |
15862-18-7 | |
Record name | 5,5'-DIBROMO-2,2'-BIPYRIDINE | |
Source | EPA DSSTox | |
URL | https://comptox.epa.gov/dashboard/DTXSID90447824 | |
Description | DSSTox provides a high quality public chemistry resource for supporting improved predictive toxicology. | |
Record name | 5,5'-Dibromo-2,2'-bipyridyl | |
Source | European Chemicals Agency (ECHA) | |
URL | https://echa.europa.eu/information-on-chemicals | |
Description | The European Chemicals Agency (ECHA) is an agency of the European Union which is the driving force among regulatory authorities in implementing the EU's groundbreaking chemicals legislation for the benefit of human health and the environment as well as for innovation and competitiveness. | |
Explanation | Use of the information, documents and data from the ECHA website is subject to the terms and conditions of this Legal Notice, and subject to other binding limitations provided for under applicable law, the information, documents and data made available on the ECHA website may be reproduced, distributed and/or used, totally or in part, for non-commercial purposes provided that ECHA is acknowledged as the source: "Source: European Chemicals Agency, http://echa.europa.eu/". Such acknowledgement must be included in each copy of the material. ECHA permits and encourages organisations and individuals to create links to the ECHA website under the following cumulative conditions: Links can only be made to webpages that provide a link to the Legal Notice page. | |
Synthesis routes and methods
Procedure details
Retrosynthesis Analysis
AI-Powered Synthesis Planning: Our tool employs the Template_relevance Pistachio, Template_relevance Bkms_metabolic, Template_relevance Pistachio_ringbreaker, Template_relevance Reaxys, Template_relevance Reaxys_biocatalysis model, leveraging a vast database of chemical reactions to predict feasible synthetic routes.
One-Step Synthesis Focus: Specifically designed for one-step synthesis, it provides concise and direct routes for your target compounds, streamlining the synthesis process.
Accurate Predictions: Utilizing the extensive PISTACHIO, BKMS_METABOLIC, PISTACHIO_RINGBREAKER, REAXYS, REAXYS_BIOCATALYSIS database, our tool offers high-accuracy predictions, reflecting the latest in chemical research and data.
Strategy Settings
Precursor scoring | Relevance Heuristic |
---|---|
Min. plausibility | 0.01 |
Model | Template_relevance |
Template Set | Pistachio/Bkms_metabolic/Pistachio_ringbreaker/Reaxys/Reaxys_biocatalysis |
Top-N result to add to graph | 6 |
Feasible Synthetic Routes
A: 5,5'-Dibromo-2,2'-bipyridine (also referred to as dbbpy in some papers) is an organic compound with the molecular formula C10H6Br2N2. [] Its molecular weight is 314.0 g/mol. [] The molecule exhibits an anti coplanar conformation of the bipyridine moiety. [] Spectroscopic data such as 1H NMR, IR, and UV-Vis are commonly used to characterize synthesized compounds incorporating this compound. [, , , , , , ]
ANone: Several synthetic routes exist:
- Stille Coupling: This method uses 2,5-dibromopyridine and hexamethylditin in the presence of a palladium catalyst like [Pd(PPh3)4]. [] Another approach couples 2,5-dibromopyridine with 5-bromo-2-trialkylstannylpyridines using palladium catalysts. []
- Reductive Symmetric Coupling: This method utilizes hexa-n-butyldistannane to couple 2,5-dibromopyridine. []
- Palladium-Catalyzed Dimerization: this compound can be unexpectedly obtained as a byproduct in the Heck reaction of 2,5-dibromopyridine with alkenes, likely through a palladium-catalyzed dimerization pathway. []
ANone: this compound is primarily used as a versatile building block in organic synthesis, particularly for:
- Ligand Synthesis: It serves as a precursor for various bidentate N-donor ligands used in coordination chemistry. These ligands are often employed in the synthesis of metal complexes with applications in catalysis, photochemistry, and materials science. [, , , , , , , ]
- Polymer Synthesis: The bromine atoms provide handles for further functionalization via cross-coupling reactions, such as the Suzuki and Sonogashira couplings, enabling the incorporation of the bipyridine unit into polymers. These polymers find applications in materials science, including light-emitting materials and porous polymers for CO2 capture. [, , , , ]
- Molecular Rod Synthesis: Its rigid structure makes it a suitable building block for constructing molecular rods, which are of interest in supramolecular chemistry and nanotechnology. []
ANone: Metal complexes incorporating ligands derived from this compound demonstrate catalytic activity in various reactions, including:
- Epoxidation: Dioxomolybdenum(VI) complexes with this compound and other substituted bipyridine ligands exhibit high activity and selectivity in the epoxidation of cyclooctene using tert-butyl hydroperoxide as the oxidant. []
- CO2 Fixation: Porous hybrid polymers incorporating this compound and coordinated ZnBr2 show enhanced heterogeneous catalytic activity in the cycloaddition of CO2 with epoxides, producing cyclic carbonates under mild, co-catalyst-free conditions. []
ANone: The structure of this compound significantly impacts its reactivity and the properties of its derivatives:
- Bromine Substituents: The bromine atoms at the 5 and 5' positions are susceptible to various cross-coupling reactions, enabling the introduction of diverse functional groups and the construction of complex molecular architectures. [, , , , , , , ]
- Bipyridine Core: The bipyridine core acts as a strong chelating ligand for various metal ions, influencing the electronic properties and reactivity of the resulting metal complexes. The nature of the substituents on the bipyridine ring can further modulate these properties, affecting the complex's catalytic activity, redox potential, and photophysical behavior. [, , , , , , , ]
- Planar Conformation: The planar conformation of the bipyridine unit contributes to the rigidity of this compound and its derivatives. This rigidity can impact the packing of molecules in the solid state and influence the morphology of polymers incorporating this unit. []
ANone: Computational chemistry plays a crucial role in studying this compound and its derivatives:
- DFT Calculations: Density Functional Theory calculations help understand the electronic structure, stability, and reactivity of metal complexes with this compound-derived ligands. These calculations can provide insights into the catalytic mechanisms and predict the properties of new complexes. []
ANone: A variety of analytical methods are employed for the characterization of this compound and its derivatives:
- Spectroscopy: 1H NMR, IR, and UV-Vis spectroscopy are commonly used to confirm the structure and investigate the electronic properties of synthesized compounds. [, , , , , , ]
- X-ray Crystallography: This technique provides detailed information about the solid-state structure of this compound and its derivatives, including bond lengths, angles, and molecular packing. [, , ]
- Electrochemistry: Cyclic voltammetry helps study the redox behavior of metal complexes containing this compound-derived ligands, providing information about their electrochemical properties and potential applications. []
Disclaimer and Information on In-Vitro Research Products
Please be aware that all articles and product information presented on BenchChem are intended solely for informational purposes. The products available for purchase on BenchChem are specifically designed for in-vitro studies, which are conducted outside of living organisms. In-vitro studies, derived from the Latin term "in glass," involve experiments performed in controlled laboratory settings using cells or tissues. It is important to note that these products are not categorized as medicines or drugs, and they have not received approval from the FDA for the prevention, treatment, or cure of any medical condition, ailment, or disease. We must emphasize that any form of bodily introduction of these products into humans or animals is strictly prohibited by law. It is essential to adhere to these guidelines to ensure compliance with legal and ethical standards in research and experimentation.