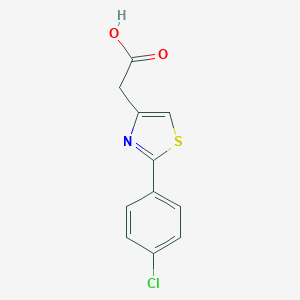
Fenclozic acid
Overview
Description
Fenclozic acid, also known as [2-(4-chlorophenyl)-1,3-thiazol-4-yl]acetic acid, is a carboxylic acid non-steroidal anti-inflammatory drug (NSAID) that emerged in the late 1960s. It demonstrated potent anti-inflammatory, antipyretic, and analgesic properties. it was withdrawn from clinical use in 1970 due to hepatotoxicity observed in clinical trials .
Mechanism of Action
Fenclozic acid, also known as 2-[2-(4-chlorophenyl)-1,3-thiazol-4-yl]acetic acid, is a compound that was initially developed as a promising non-steroidal anti-inflammatory drug (NSAID) in the 1960s .
Target of Action
This compound is a COX inhibitor . Cyclooxygenases (COX) are key enzymes in the biosynthesis of prostanoids, including prostaglandins, prostacyclin, and thromboxane, which play a significant role in inflammation, pain, and fever.
Mode of Action
As a COX inhibitor, this compound works by blocking the COX enzymes, thereby reducing the production of prostanoids. This leads to decreased inflammation, pain, and fever .
Biochemical Pathways
This compound undergoes extensive metabolism involving both oxidation and conjugation . The formation of glutathione adducts/conjugates provides evidence for the production of reactive metabolites . The production of other classes of reactive metabolites includes acyl-glucuronides, and the biosynthesis of acyl carnitine, taurine, glutamine, and glycine conjugates via potentially reactive acyl-CoA intermediates .
Pharmacokinetics
The pharmacokinetic properties of this compound have been established in several animal species and humans . The compound is restricted mainly within the central compartment, with the volume of which is less than 20% of the body weight . The biological half-life of this compound varies between species, ranging from 3 hours in monkeys to 118 hours in horses . In guinea pigs, rats, dogs, and humans, the half-life is in the range of 26-31 hours .
Result of Action
This compound demonstrated potent anti-inflammatory, antipyretic, and analgesic properties . It was withdrawn from clinical development in 1971 due to hepatotoxicity observed in clinical trials . The hepatotoxicity is suggested to be due to Phase 1 bioactivation, leading to the formation of reactive metabolites .
Action Environment
The action of this compound can be influenced by various environmental factors. For instance, the presence of NADPH in liver microsomes has been shown to result in time-dependent covalent binding to protein . Moreover, the formation of reactive metabolites and their subsequent covalent binding to proteins can be influenced by the specific metabolic environment in different species .
Biochemical Analysis
Biochemical Properties
Fenclozic acid’s metabolism is extensive, particularly involving the carboxylic acid-containing side chain . It undergoes biotransformation via both oxidative and conjugative routes . The oxidative metabolites detected include a variety of hydroxylations as well as cysteinyl-, N-acetylcysteinyl-, and cysteinylglycine metabolites . These metabolites result from the formation of glutathione adducts/conjugates, providing evidence for the production of reactive metabolites .
Cellular Effects
This compound has been shown to cause acute centrilobular hepatocellular necrosis in mice . No other regions of the liver were affected . In vitro studies have shown that this compound does not cause P450-dependent and P450-independent cytotoxicity to THLE cell lines .
Molecular Mechanism
The molecular mechanism of this compound involves the formation of electrophilic reactive metabolites (RMs), potentially unique to humans . The production of other classes of RMs includes acyl-glucuronides, and the biosynthesis of acyl carnitine, taurine, glutamine, and glycine conjugates via potentially reactive acyl-CoA intermediates .
Temporal Effects in Laboratory Settings
Following oral administration of this compound, whole body autoradiography showed distribution into all tissues except the brain, with radioactivity still detectable in blood, kidney, and liver at 72 hours post-dose .
Dosage Effects in Animal Models
The effects of this compound were investigated following single oral doses of 10 mg/kg to normal and bile duct-cannulated male C57BL/6J mice . The study did not provide specific details on the effects of different dosages.
Metabolic Pathways
This compound is involved in a number of metabolic processes. It undergoes extensive metabolism that involves biotransformations via both oxidation and conjugation . The oxidative metabolites detected include a variety of hydroxylations as well as cysteinyl-, N-acetylcysteinyl-, and cysteinylglycine metabolites .
Transport and Distribution
This compound is distributed into all tissues except the brain . The majority of the this compound-related material recovered was found in the urine/aqueous cage wash (49%), while a smaller portion (13%) was eliminated via the feces .
Subcellular Localization
Given its extensive metabolism and distribution into various tissues , it can be inferred that this compound may localize in various subcellular compartments depending on its metabolic and functional requirements.
Preparation Methods
Synthetic Routes and Reaction Conditions: The synthesis of fenclozic acid involves the reaction of 4-chlorobenzaldehyde with thiourea to form 2-(4-chlorophenyl)-1,3-thiazole. This intermediate is then reacted with chloroacetic acid to yield this compound .
Industrial Production Methods: the general synthetic route involves standard organic synthesis techniques, including condensation and substitution reactions .
Chemical Reactions Analysis
Types of Reactions: Fenclozic acid undergoes various chemical reactions, including:
Oxidation: It can be oxidized to form corresponding sulfoxides and sulfones.
Reduction: Reduction reactions can convert it to its corresponding alcohols.
Substitution: It can undergo nucleophilic substitution reactions, particularly at the thiazole ring
Common Reagents and Conditions:
Oxidation: Common oxidizing agents include hydrogen peroxide and peracids.
Reduction: Reducing agents such as lithium aluminum hydride (LiAlH4) are used.
Substitution: Nucleophiles like amines and thiols are commonly employed
Major Products:
Oxidation: Sulfoxides and sulfones.
Reduction: Alcohols.
Substitution: Various substituted thiazole derivatives
Scientific Research Applications
Fenclozic acid has been used in various scientific research applications, including:
Chemistry: As a model compound to study the reactivity of carboxylic acids and thiazole derivatives.
Biology: To investigate the metabolic pathways and toxicity mechanisms in hepatocytes.
Medicine: Initially explored as an alternative to high-dose aspirin for treating rheumatoid arthritis.
Industry: Limited industrial applications due to its withdrawal, but it has been used in research to understand drug metabolism and toxicity
Comparison with Similar Compounds
Aspirin: Another carboxylic acid NSAID with similar anti-inflammatory and analgesic properties.
Ibuprofen: A widely used NSAID with a similar mechanism of action.
Naproxen: Another NSAID with similar therapeutic effects.
Uniqueness: Fenclozic acid is unique due to its thiazole ring structure, which is not commonly found in other NSAIDs. This structural difference contributes to its distinct pharmacokinetic and pharmacodynamic properties .
Properties
IUPAC Name |
2-[2-(4-chlorophenyl)-1,3-thiazol-4-yl]acetic acid | |
---|---|---|
Source | PubChem | |
URL | https://pubchem.ncbi.nlm.nih.gov | |
Description | Data deposited in or computed by PubChem | |
InChI |
InChI=1S/C11H8ClNO2S/c12-8-3-1-7(2-4-8)11-13-9(6-16-11)5-10(14)15/h1-4,6H,5H2,(H,14,15) | |
Source | PubChem | |
URL | https://pubchem.ncbi.nlm.nih.gov | |
Description | Data deposited in or computed by PubChem | |
InChI Key |
APBSKHYXXKHJFK-UHFFFAOYSA-N | |
Source | PubChem | |
URL | https://pubchem.ncbi.nlm.nih.gov | |
Description | Data deposited in or computed by PubChem | |
Canonical SMILES |
C1=CC(=CC=C1C2=NC(=CS2)CC(=O)O)Cl | |
Source | PubChem | |
URL | https://pubchem.ncbi.nlm.nih.gov | |
Description | Data deposited in or computed by PubChem | |
Molecular Formula |
C11H8ClNO2S | |
Source | PubChem | |
URL | https://pubchem.ncbi.nlm.nih.gov | |
Description | Data deposited in or computed by PubChem | |
DSSTOX Substance ID |
DTXSID4046157 | |
Record name | Fenclozic acid | |
Source | EPA DSSTox | |
URL | https://comptox.epa.gov/dashboard/DTXSID4046157 | |
Description | DSSTox provides a high quality public chemistry resource for supporting improved predictive toxicology. | |
Molecular Weight |
253.71 g/mol | |
Source | PubChem | |
URL | https://pubchem.ncbi.nlm.nih.gov | |
Description | Data deposited in or computed by PubChem | |
CAS No. |
17969-20-9 | |
Record name | Fenclozic acid | |
Source | CAS Common Chemistry | |
URL | https://commonchemistry.cas.org/detail?cas_rn=17969-20-9 | |
Description | CAS Common Chemistry is an open community resource for accessing chemical information. Nearly 500,000 chemical substances from CAS REGISTRY cover areas of community interest, including common and frequently regulated chemicals, and those relevant to high school and undergraduate chemistry classes. This chemical information, curated by our expert scientists, is provided in alignment with our mission as a division of the American Chemical Society. | |
Explanation | The data from CAS Common Chemistry is provided under a CC-BY-NC 4.0 license, unless otherwise stated. | |
Record name | Fenclozic acid [INN:BAN] | |
Source | ChemIDplus | |
URL | https://pubchem.ncbi.nlm.nih.gov/substance/?source=chemidplus&sourceid=0017969209 | |
Description | ChemIDplus is a free, web search system that provides access to the structure and nomenclature authority files used for the identification of chemical substances cited in National Library of Medicine (NLM) databases, including the TOXNET system. | |
Record name | Fenclozic acid | |
Source | EPA DSSTox | |
URL | https://comptox.epa.gov/dashboard/DTXSID4046157 | |
Description | DSSTox provides a high quality public chemistry resource for supporting improved predictive toxicology. | |
Record name | FENCLOZIC ACID | |
Source | FDA Global Substance Registration System (GSRS) | |
URL | https://gsrs.ncats.nih.gov/ginas/app/beta/substances/58SRQ4DV53 | |
Description | The FDA Global Substance Registration System (GSRS) enables the efficient and accurate exchange of information on what substances are in regulated products. Instead of relying on names, which vary across regulatory domains, countries, and regions, the GSRS knowledge base makes it possible for substances to be defined by standardized, scientific descriptions. | |
Explanation | Unless otherwise noted, the contents of the FDA website (www.fda.gov), both text and graphics, are not copyrighted. They are in the public domain and may be republished, reprinted and otherwise used freely by anyone without the need to obtain permission from FDA. Credit to the U.S. Food and Drug Administration as the source is appreciated but not required. | |
Retrosynthesis Analysis
AI-Powered Synthesis Planning: Our tool employs the Template_relevance Pistachio, Template_relevance Bkms_metabolic, Template_relevance Pistachio_ringbreaker, Template_relevance Reaxys, Template_relevance Reaxys_biocatalysis model, leveraging a vast database of chemical reactions to predict feasible synthetic routes.
One-Step Synthesis Focus: Specifically designed for one-step synthesis, it provides concise and direct routes for your target compounds, streamlining the synthesis process.
Accurate Predictions: Utilizing the extensive PISTACHIO, BKMS_METABOLIC, PISTACHIO_RINGBREAKER, REAXYS, REAXYS_BIOCATALYSIS database, our tool offers high-accuracy predictions, reflecting the latest in chemical research and data.
Strategy Settings
Precursor scoring | Relevance Heuristic |
---|---|
Min. plausibility | 0.01 |
Model | Template_relevance |
Template Set | Pistachio/Bkms_metabolic/Pistachio_ringbreaker/Reaxys/Reaxys_biocatalysis |
Top-N result to add to graph | 6 |
Feasible Synthetic Routes
Disclaimer and Information on In-Vitro Research Products
Please be aware that all articles and product information presented on BenchChem are intended solely for informational purposes. The products available for purchase on BenchChem are specifically designed for in-vitro studies, which are conducted outside of living organisms. In-vitro studies, derived from the Latin term "in glass," involve experiments performed in controlled laboratory settings using cells or tissues. It is important to note that these products are not categorized as medicines or drugs, and they have not received approval from the FDA for the prevention, treatment, or cure of any medical condition, ailment, or disease. We must emphasize that any form of bodily introduction of these products into humans or animals is strictly prohibited by law. It is essential to adhere to these guidelines to ensure compliance with legal and ethical standards in research and experimentation.