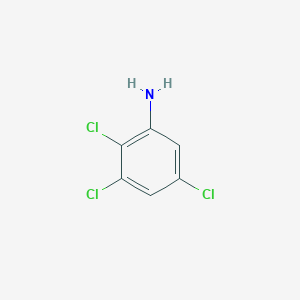
2,3,5-Trichloroaniline
Overview
Description
2,3,5-Trichloroaniline is an organic compound with the chemical formula C6H4Cl3N. It is a derivative of aniline, where three hydrogen atoms on the benzene ring are replaced by chlorine atoms at the 2, 3, and 5 positions. This compound is a colorless crystalline solid with a pungent odor and is poorly soluble in water but easily soluble in organic solvents .
Mechanism of Action
Target of Action
It’s known that chloroanilines, the class of compounds to which 2,3,5-trichloroaniline belongs, are widely used in the manufacture of drugs, pesticides, and industrial intermediates .
Mode of Action
Studies on related compounds suggest that bioactivation of chloroanilines to toxic metabolites by cytochrome p450 (cyp), cyclooxygenase, and/or peroxidase contributes to their toxicity .
Biochemical Pathways
The biotransformation of chloroanilines, including this compound, involves several biochemical pathways. These include acetylation, N-oxidation, and aromatic ring oxidation . The exact nature and sequence of these reactions, as well as their downstream effects, are subjects of ongoing research.
Pharmacokinetics
Studies on related trichloroanilines in fish suggest that biotransformation plays a significant role in their elimination .
Result of Action
Related trichloroanilines have been shown to induce cytotoxicity in isolated renal cortical cells, as evidenced by increased lactate dehydrogenase (ldh) release .
Action Environment
Environmental factors can influence the action, efficacy, and stability of this compound. For instance, dust formation should be avoided, and adequate ventilation should be ensured when handling this compound .
Biochemical Analysis
Biochemical Properties
2,3,5-Trichloroaniline plays a significant role in various biochemical reactions. It interacts with enzymes such as cytochrome P450, which is involved in its biotransformation. The compound undergoes N-acetylation, a process facilitated by N-acetyltransferase enzymes, leading to the formation of acetylated metabolites . These interactions are essential for the compound’s metabolism and detoxification.
Cellular Effects
This compound affects various cell types and cellular processes. It has been shown to induce cytotoxicity in renal cortical cells, leading to increased lactate dehydrogenase release . The compound influences cell signaling pathways, gene expression, and cellular metabolism by generating reactive oxygen species (ROS) and causing oxidative stress . These effects can disrupt normal cell function and lead to cellular damage.
Molecular Mechanism
The molecular mechanism of this compound involves its interaction with biomolecules and enzymes. The compound binds to cytochrome P450 enzymes, leading to its bioactivation into toxic metabolites . This process generates ROS, which can cause oxidative damage to cellular components. Additionally, this compound inhibits certain enzymes, affecting their normal function and leading to altered gene expression .
Temporal Effects in Laboratory Settings
In laboratory settings, the effects of this compound change over time. The compound’s stability and degradation are influenced by factors such as temperature and pH . Long-term exposure to this compound can result in persistent cellular damage and altered cellular function. Studies have shown that the compound’s cytotoxic effects increase with prolonged exposure .
Dosage Effects in Animal Models
The effects of this compound vary with different dosages in animal models. At low doses, the compound may cause mild cytotoxicity, while higher doses can lead to severe toxic effects, including nephrotoxicity . Threshold effects have been observed, where a certain dosage level triggers significant adverse effects. High doses of this compound can result in oxidative stress, organ damage, and altered metabolic processes .
Metabolic Pathways
This compound is involved in several metabolic pathways. It undergoes biotransformation by cytochrome P450 enzymes, leading to the formation of reactive metabolites . These metabolites can further interact with other enzymes and cofactors, affecting metabolic flux and metabolite levels. The compound’s metabolism is crucial for its detoxification and elimination from the body .
Transport and Distribution
Within cells and tissues, this compound is transported and distributed through various mechanisms. It can interact with transporters and binding proteins, influencing its localization and accumulation . The compound’s distribution is affected by its hydrophobicity, allowing it to partition into lipid-rich compartments. This property plays a role in its bioaccumulation and potential toxicity .
Subcellular Localization
This compound exhibits specific subcellular localization, which affects its activity and function. The compound can localize to organelles such as mitochondria and endoplasmic reticulum, where it exerts its effects . Targeting signals and post-translational modifications may direct this compound to specific compartments, influencing its interactions with biomolecules and its overall impact on cellular processes .
Preparation Methods
Synthetic Routes and Reaction Conditions: 2,3,5-Trichloroaniline can be synthesized through a multi-step reaction process. One common method involves the reaction of phenyl magnesium bromide with ethyl bromide to prepare dibromoaniline. This dibromoaniline is then reacted with magnesium chloride in dichloromethane to obtain dichloroaniline. Finally, further chlorination of dichloroaniline yields this compound .
Industrial Production Methods: In industrial settings, this compound is often produced by the chlorination of aniline. This process involves the reaction of aniline with chlorine gas in the presence of a catalyst, typically in an anhydrous solution of carbon tetrachloride. The product precipitates as a white solid, which is then purified .
Chemical Reactions Analysis
Types of Reactions: 2,3,5-Trichloroaniline undergoes various chemical reactions, including:
Oxidation: This compound can be oxidized to form corresponding nitro compounds.
Reduction: It can be reduced to form amines.
Substitution: It undergoes nucleophilic substitution reactions where the chlorine atoms can be replaced by other substituents.
Common Reagents and Conditions:
Oxidation: Common oxidizing agents include potassium permanganate and nitric acid.
Reduction: Reducing agents such as hydrogen gas in the presence of a palladium catalyst are used.
Substitution: Nucleophiles like sodium hydroxide or ammonia can be used under basic conditions.
Major Products Formed:
Oxidation: Nitro derivatives.
Reduction: Amines.
Substitution: Various substituted anilines depending on the nucleophile used
Scientific Research Applications
2,3,5-Trichloroaniline has several applications in scientific research:
Chemistry: It is used as an intermediate in the synthesis of dyes, pigments, and other organic compounds.
Comparison with Similar Compounds
- 2,4,6-Trichloroaniline
- 2,4,5-Trichloroaniline
- 3,4,5-Trichloroaniline
Comparison:
- 2,4,6-Trichloroaniline: Similar in structure but differs in the position of chlorine atoms. It is also used in the synthesis of dyes and pigments.
- 2,4,5-Trichloroaniline: Another isomer with different chlorine positions, used in herbicide production.
- 3,4,5-Trichloroaniline: Known for its nephrotoxic effects and studied for its role in renal biotransformation .
2,3,5-Trichloroaniline is unique due to its specific chlorine substitution pattern, which influences its chemical reactivity and applications in various fields.
Properties
IUPAC Name |
2,3,5-trichloroaniline | |
---|---|---|
Source | PubChem | |
URL | https://pubchem.ncbi.nlm.nih.gov | |
Description | Data deposited in or computed by PubChem | |
InChI |
InChI=1S/C6H4Cl3N/c7-3-1-4(8)6(9)5(10)2-3/h1-2H,10H2 | |
Source | PubChem | |
URL | https://pubchem.ncbi.nlm.nih.gov | |
Description | Data deposited in or computed by PubChem | |
InChI Key |
MOTBXEPLFOLWHZ-UHFFFAOYSA-N | |
Source | PubChem | |
URL | https://pubchem.ncbi.nlm.nih.gov | |
Description | Data deposited in or computed by PubChem | |
Canonical SMILES |
C1=C(C=C(C(=C1N)Cl)Cl)Cl | |
Source | PubChem | |
URL | https://pubchem.ncbi.nlm.nih.gov | |
Description | Data deposited in or computed by PubChem | |
Molecular Formula |
C6H4Cl3N | |
Source | PubChem | |
URL | https://pubchem.ncbi.nlm.nih.gov | |
Description | Data deposited in or computed by PubChem | |
DSSTOX Substance ID |
DTXSID40171667 | |
Record name | 2,3,5-Trichloroaniline | |
Source | EPA DSSTox | |
URL | https://comptox.epa.gov/dashboard/DTXSID40171667 | |
Description | DSSTox provides a high quality public chemistry resource for supporting improved predictive toxicology. | |
Molecular Weight |
196.5 g/mol | |
Source | PubChem | |
URL | https://pubchem.ncbi.nlm.nih.gov | |
Description | Data deposited in or computed by PubChem | |
CAS No. |
18487-39-3 | |
Record name | 2,3,5-Trichlorobenzenamine | |
Source | CAS Common Chemistry | |
URL | https://commonchemistry.cas.org/detail?cas_rn=18487-39-3 | |
Description | CAS Common Chemistry is an open community resource for accessing chemical information. Nearly 500,000 chemical substances from CAS REGISTRY cover areas of community interest, including common and frequently regulated chemicals, and those relevant to high school and undergraduate chemistry classes. This chemical information, curated by our expert scientists, is provided in alignment with our mission as a division of the American Chemical Society. | |
Explanation | The data from CAS Common Chemistry is provided under a CC-BY-NC 4.0 license, unless otherwise stated. | |
Record name | 2,3,5-Trichloroaniline | |
Source | ChemIDplus | |
URL | https://pubchem.ncbi.nlm.nih.gov/substance/?source=chemidplus&sourceid=0018487393 | |
Description | ChemIDplus is a free, web search system that provides access to the structure and nomenclature authority files used for the identification of chemical substances cited in National Library of Medicine (NLM) databases, including the TOXNET system. | |
Record name | 2,3,5-Trichloroaniline | |
Source | EPA DSSTox | |
URL | https://comptox.epa.gov/dashboard/DTXSID40171667 | |
Description | DSSTox provides a high quality public chemistry resource for supporting improved predictive toxicology. | |
Record name | 2,3,5-trichloroaniline | |
Source | European Chemicals Agency (ECHA) | |
URL | https://echa.europa.eu/substance-information/-/substanceinfo/100.038.507 | |
Description | The European Chemicals Agency (ECHA) is an agency of the European Union which is the driving force among regulatory authorities in implementing the EU's groundbreaking chemicals legislation for the benefit of human health and the environment as well as for innovation and competitiveness. | |
Explanation | Use of the information, documents and data from the ECHA website is subject to the terms and conditions of this Legal Notice, and subject to other binding limitations provided for under applicable law, the information, documents and data made available on the ECHA website may be reproduced, distributed and/or used, totally or in part, for non-commercial purposes provided that ECHA is acknowledged as the source: "Source: European Chemicals Agency, http://echa.europa.eu/". Such acknowledgement must be included in each copy of the material. ECHA permits and encourages organisations and individuals to create links to the ECHA website under the following cumulative conditions: Links can only be made to webpages that provide a link to the Legal Notice page. | |
Record name | 2,3,5-Trichloroaniline | |
Source | FDA Global Substance Registration System (GSRS) | |
URL | https://gsrs.ncats.nih.gov/ginas/app/beta/substances/7D27GT9JNH | |
Description | The FDA Global Substance Registration System (GSRS) enables the efficient and accurate exchange of information on what substances are in regulated products. Instead of relying on names, which vary across regulatory domains, countries, and regions, the GSRS knowledge base makes it possible for substances to be defined by standardized, scientific descriptions. | |
Explanation | Unless otherwise noted, the contents of the FDA website (www.fda.gov), both text and graphics, are not copyrighted. They are in the public domain and may be republished, reprinted and otherwise used freely by anyone without the need to obtain permission from FDA. Credit to the U.S. Food and Drug Administration as the source is appreciated but not required. | |
Synthesis routes and methods I
Procedure details
Synthesis routes and methods II
Procedure details
Retrosynthesis Analysis
AI-Powered Synthesis Planning: Our tool employs the Template_relevance Pistachio, Template_relevance Bkms_metabolic, Template_relevance Pistachio_ringbreaker, Template_relevance Reaxys, Template_relevance Reaxys_biocatalysis model, leveraging a vast database of chemical reactions to predict feasible synthetic routes.
One-Step Synthesis Focus: Specifically designed for one-step synthesis, it provides concise and direct routes for your target compounds, streamlining the synthesis process.
Accurate Predictions: Utilizing the extensive PISTACHIO, BKMS_METABOLIC, PISTACHIO_RINGBREAKER, REAXYS, REAXYS_BIOCATALYSIS database, our tool offers high-accuracy predictions, reflecting the latest in chemical research and data.
Strategy Settings
Precursor scoring | Relevance Heuristic |
---|---|
Min. plausibility | 0.01 |
Model | Template_relevance |
Template Set | Pistachio/Bkms_metabolic/Pistachio_ringbreaker/Reaxys/Reaxys_biocatalysis |
Top-N result to add to graph | 6 |
Feasible Synthetic Routes
Q1: How is 2,3,5-trichloroaniline formed in the environment?
A1: this compound (2,3,5-TriCA) can be formed as an intermediate product during the microbial reductive dechlorination of higher chlorinated anilines. Research has shown that under methanogenic conditions, microorganisms can sequentially remove chlorine atoms from compounds like pentachloronitrobenzene (PCNB) and 2,3,4,5-tetrachloroaniline (2,3,4,5-TeCA). [, ] This process leads to the formation of less chlorinated anilines, including 2,3,5-TriCA, as the halogens are replaced by protons. [, ]
Q2: Can this compound be further degraded by microorganisms?
A2: Yes, research indicates that 2,3,5-TriCA can be further dechlorinated by microorganisms under methanogenic conditions. [, ] Studies have demonstrated that microbial communities can transform 2,3,5-TriCA to 3,5-dichloroaniline (3,5-diCA), highlighting the potential for complete dechlorination of these compounds in appropriate environments. [] This sequential dechlorination process is crucial for the bioremediation of contaminated sites and offers a potential pathway for the complete removal of chloroanilines from the environment. [, ]
Disclaimer and Information on In-Vitro Research Products
Please be aware that all articles and product information presented on BenchChem are intended solely for informational purposes. The products available for purchase on BenchChem are specifically designed for in-vitro studies, which are conducted outside of living organisms. In-vitro studies, derived from the Latin term "in glass," involve experiments performed in controlled laboratory settings using cells or tissues. It is important to note that these products are not categorized as medicines or drugs, and they have not received approval from the FDA for the prevention, treatment, or cure of any medical condition, ailment, or disease. We must emphasize that any form of bodily introduction of these products into humans or animals is strictly prohibited by law. It is essential to adhere to these guidelines to ensure compliance with legal and ethical standards in research and experimentation.