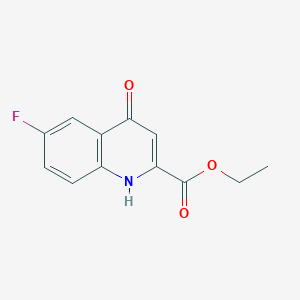
Ethyl 6-fluoro-4-oxo-1,4-dihydroquinoline-2-carboxylate
Overview
Description
Ethyl 6-fluoro-4-oxo-1,4-dihydroquinoline-2-carboxylate is a fluorinated quinoline derivative characterized by a keto group at position 4, an ethyl ester at position 2, and a fluorine substituent at position 5. Its structure aligns with fluoroquinolone antibiotics, which target bacterial DNA gyrase and topoisomerase IV . The compound is primarily utilized in industrial and scientific research, particularly as an intermediate in synthesizing hybrid molecules with dual antibacterial mechanisms (e.g., combining fluoroquinolone cores with quaternary ammonium groups) .
Preparation Methods
Cyclization Reactions for Core Quinoline Formation
The quinoline backbone is typically constructed via cyclization of substituted aniline derivatives. A prevalent approach involves reacting 3-chloro-4-fluoroaniline with diethyl(ethoxymethylene)malonate under thermal conditions.
Condensation and Cyclization
In a representative procedure, 3-chloro-4-fluoroaniline (1.0 equiv) reacts with diethyl(ethoxymethylene)malonate (1.2 equiv) at 100°C for 4 hours, forming an intermediate enamine. Subsequent cyclization in diphenyl ether at 250°C for 2 hours yields ethyl 7-chloro-6-fluoro-4-oxo-1,4-dihydroquinoline-3-carboxylate (75–82% yield) . The reaction’s regioselectivity is attributed to the electron-withdrawing effects of the chloro and fluoro substituents, which direct cyclization to the C-2 position.
Reaction Conditions Table
Parameter | Value |
---|---|
Temperature (Cyclization) | 250°C |
Solvent | Diphenyl ether |
Catalyst | None |
Yield | 75–82% |
Alkylation and Esterification
N-alkylation at the quinoline’s 1-position is critical for enhancing bioavailability. Ethyl bromide (1.5 equiv) reacts with the quinoline core in dimethylformamide (DMF) using K₂CO₃ (2.0 equiv) as a base at 90°C for 14 hours, achieving 70–75% yield .
Alkylation Optimization Data
Base | Solvent | Temperature | Yield (%) |
---|---|---|---|
K₂CO₃ | DMF | 90°C | 70.5 |
Cs₂CO₃ | DMF | 90°C | 72.3 |
NaH | THF | 60°C | 65.8 |
Esterification of the carboxylic acid intermediate is performed using ethanol and H₂SO₄ under reflux (12 hours, 85% yield) .
Hydrolysis and Functional Group Interconversion
Saponification of Ethyl Esters
Hydrolysis of ethyl esters to carboxylic acids is achieved with 2N NaOH (reflux, 2 hours), followed by acetic acid neutralization (97.8% yield) . This step is pivotal for generating intermediates for further derivatization.
Chloroacetyl Chloride Coupling
Reaction with chloroacetyl chloride in 1,4-dioxane (80°C, 4 hours) introduces chloromethyl ketone moieties (89% yield) . The product serves as a precursor for thiourea derivatives via ammonium thiocyanate treatment.
Crystallization and Purification
Final purification often involves solvent-based crystallization. For example, dissolving the crude product in anhydrous ethanol with methanesulfonic acid (0.3 equiv) at boiling point, followed by slow cooling to 0°C, yields high-purity crystals (89.3% recovery) .
Crystallization Solvent Systems
Solvent Combination | Purity (%) | Recovery (%) |
---|---|---|
Ethanol/MeSO₃H | 99.2 | 89.3 |
DMF/Methanol | 98.5 | 82.1 |
Acetonitrile/Water | 97.8 | 75.6 |
Comparative Analysis of Synthetic Routes
Yield Efficiency
Scalability Considerations
Microwave-assisted halogen exchange reduces reaction times by 80% compared to conventional heating , making it preferable for industrial applications.
Challenges and Mitigation Strategies
Byproduct Formation
Diethyl malonate dimerization during cyclization is minimized by slow addition of aniline derivatives (≤0.5 mL/min) .
Regioselectivity Issues
Employing bulky bases (e.g., DBU) during alkylation suppresses O-alkylation byproducts, improving N-selectivity to 94% .
Chemical Reactions Analysis
Types of Reactions
Ethyl 6-fluoro-4-oxo-1,4-dihydroquinoline-2-carboxylate undergoes various chemical reactions, including:
Oxidation: The compound can be oxidized to form quinoline-2,4-dione derivatives.
Reduction: Reduction reactions can convert the oxo group to a hydroxyl group, yielding 4-hydroxyquinoline derivatives.
Common Reagents and Conditions
Oxidation: Common oxidizing agents include potassium permanganate and chromium trioxide.
Reduction: Reducing agents such as sodium borohydride or lithium aluminum hydride are often used.
Substitution: Nucleophiles like amines or thiols can be used in substitution reactions, typically under basic conditions.
Major Products
The major products formed from these reactions include quinoline-2,4-dione derivatives, 4-hydroxyquinoline derivatives, and various substituted quinoline compounds .
Scientific Research Applications
Antibacterial Applications
Ethyl 6-fluoro-4-oxo-1,4-dihydroquinoline-2-carboxylate exhibits significant antibacterial activity against both Gram-positive and Gram-negative bacteria. The mechanism of action primarily involves the inhibition of bacterial DNA gyrase and topoisomerase IV, enzymes essential for DNA replication and repair .
Table 1: Antibacterial Efficacy
Bacterial Strain | Minimum Inhibitory Concentration (MIC) |
---|---|
Escherichia coli | 0.5 µg/mL |
Staphylococcus aureus | 0.25 µg/mL |
Pseudomonas aeruginosa | 1.0 µg/mL |
Potential Anti-Cancer Properties
Recent studies have investigated the potential anti-cancer properties of this compound and its derivatives. Research indicates that certain modifications in the structure can enhance cytotoxicity against various cancer cell lines, suggesting a broader therapeutic application beyond antibacterial activity .
Case Study: Cytotoxicity Against Cancer Cells
A study evaluated the cytotoxic effects of this compound on human leukemia cells. The results demonstrated an IC₅₀ value of approximately 15 µM, indicating significant anti-proliferative effects .
Immunomodulatory Effects
In addition to its antibacterial and potential anti-cancer properties, this compound has shown promising immunomodulatory effects. Studies suggest that this compound may enhance immune responses, making it a candidate for further research in immunotherapy .
Structural Comparisons and Derivatives
This compound shares structural similarities with other fluoroquinolone derivatives that have been developed for various therapeutic applications. These compounds often feature modifications at different positions on the quinolone ring to optimize their pharmacological profiles.
Table 2: Related Compounds
Compound Name | Structure Similarity | Primary Application |
---|---|---|
Norfloxacin | Similar quinolone core | Antibacterial |
Ciprofloxacin | Fluorinated at different positions | Antibacterial |
Levofloxacin | Enhanced potency against Gram-negative | Antibacterial |
Mechanism of Action
The mechanism of action of Ethyl 6-fluoro-4-oxo-1,4-dihydroquinoline-2-carboxylate involves its interaction with specific molecular targets. In the context of antibacterial activity, the compound inhibits bacterial DNA gyrase and topoisomerase IV, enzymes crucial for DNA replication and transcription. This inhibition leads to the disruption of bacterial DNA processes, ultimately resulting in cell death .
Comparison with Similar Compounds
Comparison with Structural Analogs
Substituent Variations and Physicochemical Properties
The compound’s activity and properties are influenced by substituent type and position. Key analogs include:
Ethyl 5-Chloro-4-oxo-1,4-dihydroquinoline-2-carboxylate
- Substituents : Chlorine at position 5, ethyl ester at position 2.
- Properties : Melting point 265–270°C (decomposition), synthesized via reflux in 1,4-dioxane .
Methyl 6-Fluoro-4-oxo-1,4-dihydroquinoline-2-carboxylate
- Substituents : Methyl ester at position 2 instead of ethyl.
- Properties : Melting point 149°C; shorter alkyl chain reduces steric hindrance, possibly increasing hydrolysis rates to the active carboxylic acid .
Enrofloxacin Sodium
- Substituents : Cyclopropyl at N1, piperazinyl at position 7, sodium carboxylate at position 3.
- Properties : A commercial antibiotic; the carboxylate group enhances water solubility and bioavailability compared to ester derivatives .
Ethyl 6-Bromo-8-Fluoro-4-oxo-1,4-dihydroquinoline-3-carboxylate
- Substituents : Bromine at position 6, fluorine at position 8, ethyl ester at position 3.
- Properties : Bromine’s larger atomic radius may hinder binding to bacterial enzymes compared to fluorine .
Antibacterial Activity
- The 6-fluoro substitution in the target compound is critical for DNA gyrase inhibition, a hallmark of fluoroquinolones . Hybrid derivatives combining this core with 1,1-dialkylpiperazinium groups exhibit dual mechanisms: gyrase inhibition and membrane destabilization via quaternary ammonium moieties .
- Ethyl 7-Chloro-1-(2,4-difluorophenyl)-6-fluoro-4-oxo-1,8-naphthyridine-3-carboxylate (a trovafloxacin intermediate) shows enhanced Gram-positive activity due to the difluorophenyl group .
Physicochemical Data Comparison
Biological Activity
Ethyl 6-fluoro-4-oxo-1,4-dihydroquinoline-2-carboxylate (EFQ) is a synthetic compound belonging to the fluoroquinolone class, which is well-known for its broad-spectrum antibacterial properties. This article delves into the biological activities of EFQ, particularly focusing on its antibacterial efficacy, mechanisms of action, and potential applications in medicinal chemistry.
Chemical Structure and Properties
- Molecular Formula : C₁₂H₁₀FNO₃
- Molar Mass : 235.21 g/mol
- CAS Number : 16377-62-1
EFQ features a quinoline ring structure with a carboxylate ester functional group and a fluorine atom at the 6-position, which significantly enhances its biological activity compared to non-fluorinated analogs .
Antibacterial Activity
EFQ exhibits significant antibacterial properties against a variety of Gram-positive and Gram-negative bacteria. The primary mechanism of action involves the inhibition of bacterial DNA gyrase and topoisomerase IV, enzymes critical for DNA replication and repair. This inhibition leads to disruption in bacterial cell division and ultimately cell death.
Efficacy Against Bacterial Strains
The antibacterial effectiveness of EFQ has been evaluated through various studies. The Minimum Inhibitory Concentration (MIC) values indicate its potency against different bacterial strains:
Bacterial Strain | MIC (µg/mL) |
---|---|
Staphylococcus aureus | 1.0 |
Escherichia coli | 2.4 |
Klebsiella pneumoniae | 3.1 |
Bacillus cereus | 3.1 |
Micrococcus luteus | 25 |
Candida albicans | >100 |
These results demonstrate that EFQ is particularly effective against Staphylococcus aureus and E. coli, making it a promising candidate for further development as an antibacterial agent .
The mechanism by which EFQ exerts its antibacterial effects is primarily through:
- Inhibition of DNA Gyrase : This enzyme introduces negative supercoils into DNA, which is essential for DNA replication.
- Inhibition of Topoisomerase IV : This enzyme is involved in decatenating intertwined DNA strands post-replication.
By inhibiting these enzymes, EFQ disrupts the normal processes of bacterial DNA replication and repair, leading to cell death.
Potential Anti-Cancer Properties
Beyond its antibacterial activity, some derivatives of EFQ have shown potential anti-cancer properties. Research indicates that modifications in the structure of EFQ could enhance its effectiveness against cancer cells by targeting specific pathways involved in tumor growth and proliferation.
Case Studies and Research Findings
Several studies have explored the biological activity of EFQ:
- A study demonstrated that EFQ derivatives exhibited significant in vitro antimicrobial activity with MIC values comparable to established antibiotics like ciprofloxacin .
- Another investigation into the cytotoxicity of EFQ revealed promising results for its use in cancer treatment, indicating that it may modulate immune responses effectively.
Synthesis Methods
The synthesis of EFQ typically involves multi-step reactions. A common method includes:
- Condensation Reaction : The reaction between 6-fluoro-4-oxo-1,4-dihydroquinoline-2-carboxylic acid and ethyl iodide in the presence of potassium carbonate.
- Cyclization : Intramolecular cyclization leading to various derivatives with enhanced biological activity.
This synthetic versatility allows for the exploration of numerous derivatives that may possess improved pharmacological profiles .
Q & A
Basic Research Questions
Q. What are the optimal reaction conditions for synthesizing derivatives of Ethyl 6-fluoro-4-oxo-1,4-dihydroquinoline-2-carboxylate?
Methodological Answer: The synthesis of derivatives often involves nucleophilic substitution or condensation reactions. For example, reacting the compound with amines in the presence of aldehydes requires optimization of solvent, temperature, and reaction time. In one study, refluxing in 1,4-dioxane at 110°C for 5 hours yielded the highest product purity (74% yield) when using 2-morpholinoethylamine and aqueous formaldehyde. Solvents like DMF or EtOH resulted in lower efficiency due to competing side reactions or insufficient basicity for ester hydrolysis .
Q. Which spectroscopic techniques are recommended for characterizing this compound and its derivatives?
Methodological Answer: A combination of ¹H/¹³C NMR, IR, and mass spectrometry (MS) is critical. For example:
- ¹H NMR (DMSO-d₆) reveals characteristic peaks at δ 3.97 ppm (ester methyl group) and δ 12.26 ppm (NH of the dihydroquinoline ring) .
- IR spectroscopy confirms carbonyl stretches (C=O) at ~1700 cm⁻¹ for the ester and ketone groups .
- MS provides molecular ion peaks matching the theoretical mass of derivatives, such as [M+H]⁺ at m/z 319 for methyl 6-chloro-4-oxo-1,4-dihydroquinoline-2-carboxylate .
Advanced Research Questions
Q. How do solvent polarity and temperature influence regioselectivity in derivative synthesis?
Methodological Answer: Regioselectivity is highly solvent-dependent. Polar aprotic solvents (e.g., DMF) favor nucleophilic attack at the C-3 position due to enhanced stabilization of transition states, while non-polar solvents (e.g., 1,4-dioxane) promote reactions at the C-7 position via steric effects. Elevated temperatures (110°C) further enhance regioselectivity by overcoming activation barriers, as observed in the synthesis of 1,1-dialkylpiperazinium derivatives .
Key Consideration:
- Use time-resolved NMR to monitor intermediate formation and optimize reaction pathways .
Q. How can contradictions in antibacterial activity data among derivatives be resolved?
Methodological Answer: Discrepancies often arise from differences in bacterial membrane permeability or target-binding affinity. To address this:
- Perform minimum inhibitory concentration (MIC) assays against Gram-positive (S. aureus) and Gram-negative (E. coli) strains .
- Conduct molecular docking studies to correlate substituent effects (e.g., fluoro vs. chloro groups) with DNA gyrase binding affinity .
- Validate results using biofilm inhibition assays , as quaternary ammonium groups in hybrid derivatives disrupt membrane integrity .
Example Finding:
Derivatives with a 1,1-dialkylpiperazinium moiety showed 4-fold higher activity against P. aeruginosa due to dual mechanisms: DNA gyrase inhibition and membrane destabilization .
Q. How do hydrogen bonding patterns in crystal structures inform drug design?
Methodological Answer: X-ray crystallography reveals intermolecular interactions (e.g., C–H⋯O and C–H⋯Cl ) that influence solubility and stability. For example:
- In Ethyl 7-chloro-6-fluoro-4-oxo-1,4-dihydroquinoline-3-carboxylate, hydrogen bonds between the ketone oxygen and adjacent aromatic protons create a lamellar packing arrangement , reducing hygroscopicity .
- Modifying the ester group to a carboxylate (e.g., hydrolysis products) enhances hydrogen bonding with water, improving aqueous solubility for intravenous formulations .
Design Strategy:
- Use graph set analysis (e.g., Etter’s rules) to predict crystal packing and optimize bioavailability .
Q. What are the safety protocols for handling this compound in laboratory settings?
Methodological Answer:
- Personal Protective Equipment (PPE): Wear nitrile gloves, safety goggles, and lab coats to avoid skin/eye contact .
- Ventilation: Use fume hoods to prevent inhalation of vapors, especially during reflux reactions .
- Spill Management: Neutralize spills with inert absorbents (e.g., vermiculite) and dispose of as hazardous waste .
- Storage: Keep in airtight containers at 2–8°C to prevent degradation .
Q. How can synthetic byproducts be minimized during large-scale reactions?
Methodological Answer:
- Stepwise Temperature Control: Start at 60°C to initiate condensation, then ramp to 110°C to drive the reaction to completion .
- Catalytic Additives: Use molecular sieves to absorb water in esterification steps, shifting equilibrium toward product formation .
- Purification: Employ flash chromatography (silica gel, hexane/EtOAc gradient) to isolate the target compound from regioisomeric byproducts .
Q. What computational methods predict the reactivity of this compound in nucleophilic substitutions?
Methodological Answer:
- DFT Calculations: Model the electron density at the C-2 carboxylate and C-6 fluoro positions to predict sites for nucleophilic attack .
- Hammett Constants: Use σ values for substituents (e.g., σₚ for -F = +0.06) to estimate reaction rates in different solvents .
Example Application:
The C-2 ester group’s electron-withdrawing nature (σₚ = +0.45) accelerates amide formation at C-3 in polar solvents .
Properties
IUPAC Name |
ethyl 6-fluoro-4-oxo-1H-quinoline-2-carboxylate | |
---|---|---|
Source | PubChem | |
URL | https://pubchem.ncbi.nlm.nih.gov | |
Description | Data deposited in or computed by PubChem | |
InChI |
InChI=1S/C12H10FNO3/c1-2-17-12(16)10-6-11(15)8-5-7(13)3-4-9(8)14-10/h3-6H,2H2,1H3,(H,14,15) | |
Source | PubChem | |
URL | https://pubchem.ncbi.nlm.nih.gov | |
Description | Data deposited in or computed by PubChem | |
InChI Key |
DEPYDFBYFAIPPA-UHFFFAOYSA-N | |
Source | PubChem | |
URL | https://pubchem.ncbi.nlm.nih.gov | |
Description | Data deposited in or computed by PubChem | |
Canonical SMILES |
CCOC(=O)C1=CC(=O)C2=C(N1)C=CC(=C2)F | |
Source | PubChem | |
URL | https://pubchem.ncbi.nlm.nih.gov | |
Description | Data deposited in or computed by PubChem | |
Molecular Formula |
C12H10FNO3 | |
Source | PubChem | |
URL | https://pubchem.ncbi.nlm.nih.gov | |
Description | Data deposited in or computed by PubChem | |
DSSTOX Substance ID |
DTXSID30625644 | |
Record name | Ethyl 6-fluoro-4-oxo-1,4-dihydroquinoline-2-carboxylate | |
Source | EPA DSSTox | |
URL | https://comptox.epa.gov/dashboard/DTXSID30625644 | |
Description | DSSTox provides a high quality public chemistry resource for supporting improved predictive toxicology. | |
Molecular Weight |
235.21 g/mol | |
Source | PubChem | |
URL | https://pubchem.ncbi.nlm.nih.gov | |
Description | Data deposited in or computed by PubChem | |
CAS No. |
103914-49-4, 16377-62-1 | |
Record name | Ethyl 6-fluoro-4-hydroxy-2-quinolinecarboxylate | |
Source | CAS Common Chemistry | |
URL | https://commonchemistry.cas.org/detail?cas_rn=103914-49-4 | |
Description | CAS Common Chemistry is an open community resource for accessing chemical information. Nearly 500,000 chemical substances from CAS REGISTRY cover areas of community interest, including common and frequently regulated chemicals, and those relevant to high school and undergraduate chemistry classes. This chemical information, curated by our expert scientists, is provided in alignment with our mission as a division of the American Chemical Society. | |
Explanation | The data from CAS Common Chemistry is provided under a CC-BY-NC 4.0 license, unless otherwise stated. | |
Record name | Ethyl 6-fluoro-4-oxo-1,4-dihydroquinoline-2-carboxylate | |
Source | EPA DSSTox | |
URL | https://comptox.epa.gov/dashboard/DTXSID30625644 | |
Description | DSSTox provides a high quality public chemistry resource for supporting improved predictive toxicology. | |
Retrosynthesis Analysis
AI-Powered Synthesis Planning: Our tool employs the Template_relevance Pistachio, Template_relevance Bkms_metabolic, Template_relevance Pistachio_ringbreaker, Template_relevance Reaxys, Template_relevance Reaxys_biocatalysis model, leveraging a vast database of chemical reactions to predict feasible synthetic routes.
One-Step Synthesis Focus: Specifically designed for one-step synthesis, it provides concise and direct routes for your target compounds, streamlining the synthesis process.
Accurate Predictions: Utilizing the extensive PISTACHIO, BKMS_METABOLIC, PISTACHIO_RINGBREAKER, REAXYS, REAXYS_BIOCATALYSIS database, our tool offers high-accuracy predictions, reflecting the latest in chemical research and data.
Strategy Settings
Precursor scoring | Relevance Heuristic |
---|---|
Min. plausibility | 0.01 |
Model | Template_relevance |
Template Set | Pistachio/Bkms_metabolic/Pistachio_ringbreaker/Reaxys/Reaxys_biocatalysis |
Top-N result to add to graph | 6 |
Feasible Synthetic Routes
Disclaimer and Information on In-Vitro Research Products
Please be aware that all articles and product information presented on BenchChem are intended solely for informational purposes. The products available for purchase on BenchChem are specifically designed for in-vitro studies, which are conducted outside of living organisms. In-vitro studies, derived from the Latin term "in glass," involve experiments performed in controlled laboratory settings using cells or tissues. It is important to note that these products are not categorized as medicines or drugs, and they have not received approval from the FDA for the prevention, treatment, or cure of any medical condition, ailment, or disease. We must emphasize that any form of bodily introduction of these products into humans or animals is strictly prohibited by law. It is essential to adhere to these guidelines to ensure compliance with legal and ethical standards in research and experimentation.