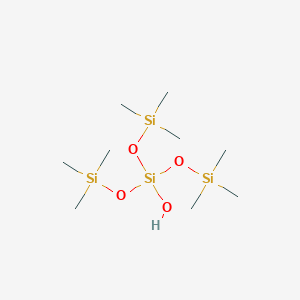
Hydroxy-tris(trimethylsilyloxy)silane
Overview
Description
Hydroxy-tris(trimethylsilyloxy)silane is an organosilicon compound with the molecular formula C₉H₂₈O₄Si₄. It is characterized by the presence of three trimethylsiloxy groups attached to a central silicon atom. This compound is known for its unique properties, including high thermal stability and hydrophobicity, making it valuable in various industrial and scientific applications.
Preparation Methods
Synthetic Routes and Reaction Conditions: The synthesis of tris(trimethylsiloxy) silanol typically involves the reaction of hexamethyldisiloxane with methanol in the presence of sulfuric acid, followed by the addition of tetramethyl orthosilicate. The reaction is carried out under controlled temperature conditions, usually between 5°C and 25°C. The final product is obtained through distillation, yielding a high-purity compound .
Industrial Production Methods: In industrial settings, the production of tris(trimethylsiloxy) silanol follows similar synthetic routes but on a larger scale. The process involves the use of large reactors and precise control of reaction conditions to ensure consistent quality and yield. The compound is then purified through distillation and other separation techniques to achieve the desired purity levels.
Chemical Reactions Analysis
Types of Reactions: Hydroxy-tris(trimethylsilyloxy)silane undergoes various chemical reactions, including:
Oxidation: The compound can be oxidized to form silanols and other silicon-containing compounds.
Reduction: It can act as a reducing agent in certain reactions, particularly in the presence of radical initiators.
Substitution: The trimethylsiloxy groups can be substituted with other functional groups under appropriate conditions.
Common Reagents and Conditions:
Oxidation: Common oxidizing agents include ozone and hydrogen peroxide.
Reduction: Radical initiators such as azo compounds and organic peroxides are used.
Substitution: Various nucleophiles can be employed to achieve substitution reactions.
Major Products Formed:
Oxidation: Silanols and disilanols.
Reduction: Reduced silicon-containing compounds.
Substitution: Compounds with different functional groups replacing the trimethylsiloxy groups
Scientific Research Applications
Hydroxy-tris(trimethylsilyloxy)silane has a wide range of applications in scientific research:
Chemistry: It is used as a reagent in organic synthesis, particularly in radical reactions and hydrosilylation processes.
Biology: The compound is employed in the modification of biomolecules and surfaces to enhance their stability and functionality.
Medicine: It is explored for its potential in drug delivery systems and as a component in medical devices.
Industry: this compound is used in the production of high-performance materials, coatings, and adhesives due to its thermal stability and hydrophobic properties
Mechanism of Action
The mechanism of action of tris(trimethylsiloxy) silanol involves its ability to participate in radical reactions and form stable intermediates. The compound can donate or accept electrons, facilitating various chemical transformations. In the presence of radical initiators, it can undergo homolytic cleavage, generating reactive species that drive the reaction forward. The molecular targets and pathways involved depend on the specific reaction and conditions employed .
Comparison with Similar Compounds
- Tris(trimethylsilyl)silane
- Chlorotris(trimethylsilyl)silane
- Triethoxysilane
- Tris(triethylsilyl)silane
Comparison: Hydroxy-tris(trimethylsilyloxy)silane is unique due to its high thermal stability and hydrophobicity compared to other similar compounds. While tris(trimethylsilyl)silane and chlorotris(trimethylsilyl)silane are also used in radical reactions, tris(trimethylsiloxy) silanol offers better stability and is less toxic. Triethoxysilane and tris(triethylsilyl)silane have different functional groups, leading to variations in reactivity and applications .
Biological Activity
Hydroxy-tris(trimethylsilyloxy)silane (HTMS) is an organosilicon compound with the molecular formula C₉H₂₈O₄Si₄. It is characterized by three trimethylsiloxy groups attached to a central silicon atom, which imparts unique properties such as high thermal stability and hydrophobicity. This compound has garnered attention in various fields, particularly in organic synthesis and biological applications. This article will delve into its biological activity, mechanisms, and potential applications based on diverse research findings.
Chemical Structure and Stability
HTMS is notable for its high thermal stability and hydrophobic characteristics , making it suitable for use in harsh environments. The presence of multiple silicon-oxygen bonds contributes to its stability, allowing it to act as a reagent in various chemical reactions, including radical reactions and hydrosilylation processes.
The biological activity of HTMS can be attributed to its ability to participate in radical reactions, forming stable intermediates. It can donate or accept electrons, facilitating various chemical transformations. In the presence of radical initiators, HTMS undergoes homolytic cleavage, generating reactive species that can interact with biological molecules . This property is particularly relevant in drug delivery systems where controlled release and interaction with biomolecules are essential.
Drug Delivery Systems
HTMS has been explored for its potential in drug delivery systems due to its ability to modify biomolecules and surfaces. Its hydrophobic nature allows it to encapsulate hydrophobic drugs effectively, enhancing their solubility and bioavailability. Studies have indicated that HTMS can improve the stability of drug formulations by forming protective siloxane layers around active pharmaceutical ingredients .
Antimicrobial Activity
Research has demonstrated that HTMS exhibits antimicrobial properties. For instance, studies involving organosilicon compounds similar to HTMS have shown effectiveness against various bacterial strains. The mechanism is believed to involve disruption of microbial cell membranes due to the hydrophobic interactions facilitated by the siloxane groups .
Case Studies
- Antimicrobial Efficacy : A study evaluated the antimicrobial activity of HTMS derivatives against common pathogens. Results indicated significant inhibition zones compared to control samples, suggesting a promising application in coatings for medical devices .
- Biocompatibility : In a study assessing biocompatibility, HTMS-modified surfaces were tested for cytotoxicity using human fibroblast cells. The results showed that HTMS did not induce significant cytotoxic effects, indicating its potential for biomedical applications .
Summary of Biological Activities
Comparison of Siloxane Compounds
Compound | Molecular Formula | Biological Activity |
---|---|---|
This compound | C₉H₂₈O₄Si₄ | Antimicrobial, drug delivery |
Tris(trimethylsiloxy)silane | C₉H₂₈O₃Si₃ | Limited biological activity |
Octamethylcyclotetrasiloxane | C₈H₂₄O₄Si₄ | Low antimicrobial activity |
Properties
IUPAC Name |
hydroxy-tris(trimethylsilyloxy)silane | |
---|---|---|
Source | PubChem | |
URL | https://pubchem.ncbi.nlm.nih.gov | |
Description | Data deposited in or computed by PubChem | |
InChI |
InChI=1S/C9H28O4Si4/c1-14(2,3)11-17(10,12-15(4,5)6)13-16(7,8)9/h10H,1-9H3 | |
Source | PubChem | |
URL | https://pubchem.ncbi.nlm.nih.gov | |
Description | Data deposited in or computed by PubChem | |
InChI Key |
ULFYRAYSKDXNLU-UHFFFAOYSA-N | |
Source | PubChem | |
URL | https://pubchem.ncbi.nlm.nih.gov | |
Description | Data deposited in or computed by PubChem | |
Canonical SMILES |
C[Si](C)(C)O[Si](O)(O[Si](C)(C)C)O[Si](C)(C)C | |
Source | PubChem | |
URL | https://pubchem.ncbi.nlm.nih.gov | |
Description | Data deposited in or computed by PubChem | |
Molecular Formula |
C9H28O4Si4 | |
Source | PubChem | |
URL | https://pubchem.ncbi.nlm.nih.gov | |
Description | Data deposited in or computed by PubChem | |
DSSTOX Substance ID |
DTXSID90375395 | |
Record name | tris(trimethylsiloxy) silanol | |
Source | EPA DSSTox | |
URL | https://comptox.epa.gov/dashboard/DTXSID90375395 | |
Description | DSSTox provides a high quality public chemistry resource for supporting improved predictive toxicology. | |
Molecular Weight |
312.66 g/mol | |
Source | PubChem | |
URL | https://pubchem.ncbi.nlm.nih.gov | |
Description | Data deposited in or computed by PubChem | |
CAS No. |
17477-97-3 | |
Record name | tris(trimethylsiloxy) silanol | |
Source | EPA DSSTox | |
URL | https://comptox.epa.gov/dashboard/DTXSID90375395 | |
Description | DSSTox provides a high quality public chemistry resource for supporting improved predictive toxicology. | |
Retrosynthesis Analysis
AI-Powered Synthesis Planning: Our tool employs the Template_relevance Pistachio, Template_relevance Bkms_metabolic, Template_relevance Pistachio_ringbreaker, Template_relevance Reaxys, Template_relevance Reaxys_biocatalysis model, leveraging a vast database of chemical reactions to predict feasible synthetic routes.
One-Step Synthesis Focus: Specifically designed for one-step synthesis, it provides concise and direct routes for your target compounds, streamlining the synthesis process.
Accurate Predictions: Utilizing the extensive PISTACHIO, BKMS_METABOLIC, PISTACHIO_RINGBREAKER, REAXYS, REAXYS_BIOCATALYSIS database, our tool offers high-accuracy predictions, reflecting the latest in chemical research and data.
Strategy Settings
Precursor scoring | Relevance Heuristic |
---|---|
Min. plausibility | 0.01 |
Model | Template_relevance |
Template Set | Pistachio/Bkms_metabolic/Pistachio_ringbreaker/Reaxys/Reaxys_biocatalysis |
Top-N result to add to graph | 6 |
Feasible Synthetic Routes
Disclaimer and Information on In-Vitro Research Products
Please be aware that all articles and product information presented on BenchChem are intended solely for informational purposes. The products available for purchase on BenchChem are specifically designed for in-vitro studies, which are conducted outside of living organisms. In-vitro studies, derived from the Latin term "in glass," involve experiments performed in controlled laboratory settings using cells or tissues. It is important to note that these products are not categorized as medicines or drugs, and they have not received approval from the FDA for the prevention, treatment, or cure of any medical condition, ailment, or disease. We must emphasize that any form of bodily introduction of these products into humans or animals is strictly prohibited by law. It is essential to adhere to these guidelines to ensure compliance with legal and ethical standards in research and experimentation.