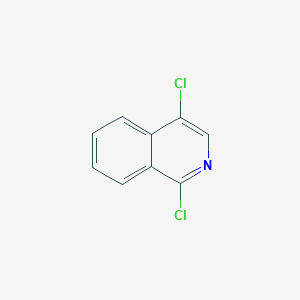
1,4-Dichloroisoquinoline
Overview
Description
1,4-Dichloroisoquinoline (CAS: 15298-58-5) is a halogenated heterocyclic compound with the molecular formula C₉H₅Cl₂N and a molecular weight of 198.05 g/mol. It is synthesized via the reaction of isocarbostyril with phosphorus pentachloride (PCl₅) at 140°C, yielding long, white needles with a melting point of 92–94°C . The compound is stored under inert atmospheres due to its reactivity and exhibits hazards including skin/eye irritation (H315, H319) and respiratory irritation (H335) . Its primary application lies in pharmaceutical synthesis, serving as a precursor for receptor ligands and bioactive derivatives .
Preparation Methods
Direct Chlorination of Isoquinoline
The direct chlorination of isoquinoline remains a foundational method for introducing chlorine atoms at the 1- and 4-positions. This approach typically employs chlorine gas (Cl₂) in the presence of Lewis acid catalysts such as iron(III) chloride (FeCl₃) or aluminum chloride (AlCl₃) . The reaction proceeds via electrophilic aromatic substitution, where the electron-deficient pyridine ring directs chlorination to specific positions.
Key Reaction Conditions:
-
Temperature: 50–70°C to balance reactivity and selectivity.
-
Catalyst Loading: 10–15 mol% FeCl₃ relative to isoquinoline.
-
Solvent: Dichloromethane or chloroform to enhance solubility.
Challenges:
-
Regioselectivity: Competing chlorination at the 1-, 3-, and 4-positions necessitates careful optimization.
-
Byproduct Formation: Over-chlorination can yield tri- or tetrachlorinated derivatives, requiring purification via fractional distillation or column chromatography .
Vilsmeier-Haack Reaction for Functionalized Isoquinolines
The Vilsmeier-Haack reaction offers a versatile route to 1,4-dichloroisoquinoline by leveraging bis(trichloromethyl) carbonate (BTC) and dimethylformamide (DMF) . This method is advantageous for its one-step protocol and high functional group tolerance.
Mechanism:
-
Formation of Chloroiminium Intermediate: DMF reacts with BTC to generate a reactive chloroiminium species.
-
Cyclization: The intermediate facilitates cyclization of phenacyl azides or analogous precursors, forming the isoquinoline core.
-
Chlorination: Concurrent chlorination at the 1- and 4-positions occurs under the reaction conditions.
Optimized Parameters:
-
Reagents: BTC (1.2 equiv), DMF (2.0 equiv).
-
Temperature: 80–100°C in toluene.
Advantages:
-
Avoids hazardous chlorine gas.
-
Compatible with electron-deficient and electron-rich substrates.
Phosphorus Oxychloride-Mediated Chlorination
Phosphorus oxychloride (POCl₃) is widely used to convert hydroxylated isoquinoline precursors into their chlorinated analogs. This method is particularly effective for synthesizing this compound from 1-hydroxy-4-chloroisoquinoline .
Procedure:
-
Substrate Preparation: 1-Hydroxy-4-chloroisoquinoline is suspended in anhydrous toluene.
-
Chlorination: POCl₃ (3.0 equiv) and catalytic DMF (0.1 equiv) are added, and the mixture is heated at 80°C for 3–5 hours.
-
Workup: The reaction is quenched with ice water, and the product is extracted with ethyl acetate.
Yield: 85–90% after recrystallization from ethanol .
Industrial Application:
-
Scalable to kilogram quantities with minimal byproducts.
-
Cost-effective compared to trifluoromethanesulfonic anhydride-based routes .
Industrial-Scale Production Methods
Large-scale synthesis prioritizes continuous flow processes to enhance efficiency and safety. A representative industrial protocol involves:
-
Continuous Chlorination: Isoquinoline and Cl₂ gas are fed into a tubular reactor with FeCl₃ catalyst at 60°C.
-
In-line Purification: The crude product undergoes fractional distillation to isolate this compound (purity >98%).
-
Waste Management: Unreacted Cl₂ is scrubbed with NaOH solution to minimize environmental impact.
Key Metrics:
Comparative Analysis of Synthetic Routes
Method | Reagents | Temperature | Yield | Purity | Scalability |
---|---|---|---|---|---|
Direct Chlorination | Cl₂, FeCl₃ | 50–70°C | 60–70% | 95% | High |
Vilsmeier-Haack | BTC, DMF | 80–100°C | 65–75% | 97% | Moderate |
POCl₃-Mediated | POCl₃, DMF | 80°C | 85–90% | 99% | High |
Industrial Flow Process | Cl₂, FeCl₃ | 60°C | 70–75% | 98% | Very High |
Trade-offs:
-
Direct Chlorination: Lower yield but cost-effective for bulk production.
-
POCl₃-Mediated: Higher yield but generates stoichiometric phosphoric acid waste.
Chemical Reactions Analysis
Types of Reactions
1,4-Dichloroisoquinoline undergoes various chemical reactions, including:
Nucleophilic Substitution: The chlorine atoms can be replaced by nucleophiles such as amines, thiols, or alkoxides.
Oxidation: The compound can be oxidized to form corresponding quinoline derivatives.
Reduction: Reduction reactions can convert this compound to its dihydro derivatives.
Common Reagents and Conditions
Nucleophilic Substitution: Reagents such as sodium amide or potassium thiolate in polar aprotic solvents like dimethyl sulfoxide.
Oxidation: Reagents like potassium permanganate or chromium trioxide in acidic conditions.
Reduction: Catalysts such as palladium on carbon in the presence of hydrogen gas.
Major Products
Nucleophilic Substitution: Produces substituted isoquinoline derivatives.
Oxidation: Forms quinoline derivatives.
Reduction: Yields dihydroisoquinoline derivatives.
Scientific Research Applications
1,4-Dichloroisoquinoline has a wide range of applications in scientific research:
Chemistry: Used as an intermediate in the synthesis of various heterocyclic compounds.
Biology: Investigated for its potential biological activities, including antimicrobial and anticancer properties.
Medicine: Explored as a precursor for the development of pharmaceutical agents.
Industry: Utilized in the production of dyes, pigments, and agrochemicals
Mechanism of Action
The mechanism of action of 1,4-Dichloroisoquinoline involves its interaction with specific molecular targets. In biological systems, it can inhibit certain enzymes or interact with DNA, leading to its antimicrobial or anticancer effects. The exact pathways and molecular targets depend on the specific application and the derivatives formed from this compound .
Comparison with Similar Compounds
Comparison with Structural Analogues
Structural Isomers: 1,7-Dichloroisoquinoline and 1,8-Dichloroisoquinoline
1,7-Dichloroisoquinoline (CAS: 70810-24-1) and 1,8-Dichloroisoquinoline are positional isomers differing in chlorine substitution on the isoquinoline ring. Key distinctions include:
- Positional differences likely alter electronic properties, affecting reactivity in substitution reactions .
- Applications: 1,8-Dichloroisoquinoline is highlighted in market analyses for niche industrial applications, though specific uses are undisclosed. In contrast, 1,4-dichloroisoquinoline is explicitly utilized in drug discovery .
Table 1: Structural Isomers of Dichloroisoquinoline
Property | This compound | 1,7-Dichloroisoquinoline | 1,8-Dichloroisoquinoline |
---|---|---|---|
CAS Number | 15298-58-5 | 70810-24-1 | Undisclosed |
Molecular Formula | C₉H₅Cl₂N | C₉H₅Cl₂N | C₉H₅Cl₂N |
Hazards | H315, H319, H335 | Harmful by inhalation | Undisclosed |
Primary Use | Pharmaceutical precursor | Undisclosed | Industrial applications |
Functionalized Analogues: 3-Chloro-1-(4-methylpiperazinyl)-1,4-dihydroisoquinoline
This derivative (C₁₄H₁₈ClN₃) incorporates a 4-methylpiperazine group at position 1 and chlorine at position 3. Key differences from this compound include:
- Synthesis: Prepared via nucleophilic substitution of 1,3-dichloroisoquinoline with N-methylpiperazine, demonstrating regioselectivity influenced by chlorine positioning .
- Applications : The piperazine moiety enhances binding to biological targets (e.g., 5-HT₃ receptors), highlighting the impact of functional groups on bioactivity .
Table 2: Comparison with Functionalized Analogues
Property | This compound | 3-Chloro-1-(4-methylpiperazinyl)-1,4-dihydroisoquinoline |
---|---|---|
Molecular Weight | 198.05 g/mol | 263.77 g/mol |
Key Functional Groups | Two chlorines at 1,4-positions | Chlorine at position 3; 4-methylpiperazine at position 1 |
Bioactivity | Precursor for ligands | Potent 5-HT₃ receptor ligand |
Sulfonamide Derivatives: 5-(1,4-Diazepan-1-ylsulfonyl)-4-methylisoquinoline
This derivative (C₁₅H₁₉N₃O₂S) features a sulfonyl-diazepane group and methyl substitution. Differences include:
- Synthesis : Introduces sulfonamide and diazepane groups via post-functionalization, increasing molecular weight to 305.40 g/mol .
- Physicochemical Properties: Higher hydrophilicity due to sulfonamide and diazepane groups, contrasting with the lipophilic nature of this compound .
Reactivity and Substitution Patterns
- This compound: The 1-chloro group is selectively hydrolyzed to form 4-chloroisocarbostyril, enabling sequential derivatization .
- 1,3-Dichloroisoquinoline: Reacts with ammonia or methylamine at the 1-position, yielding 1-amino-3-chloroisoquinoline derivatives .
Table 3: Reactivity Comparison
Compound | Reaction Partner | Product |
---|---|---|
This compound | Hydrochloric acid | 4-Chloroisocarbostyril |
1,3-Dichloroisoquinoline | N-Methylpiperazine | 1-Piperazinyl-3-chloroisoquinoline |
Biological Activity
1,4-Dichloroisoquinoline (1,4-DCI) is a compound of significant interest in medicinal chemistry due to its notable biological activities, particularly in the fields of antimicrobial and anticancer research. This article provides a comprehensive overview of the biological activity of 1,4-DCI, highlighting its mechanisms of action, experimental findings, and potential applications.
This compound is characterized by its molecular formula and a molecular weight of approximately 203.06 g/mol. It appears as a pale yellow to brown solid and is soluble in organic solvents like dimethyl sulfoxide (DMSO) but insoluble in water. The presence of chlorine substituents enhances its reactivity, making it a valuable intermediate in organic synthesis .
The biological activity of 1,4-DCI is primarily attributed to its ability to interact with specific molecular targets within biological systems. Key mechanisms include:
- Enzyme Inhibition : 1,4-DCI has been shown to inhibit plasminogen activators such as urokinase-type plasminogen activator (uPA) and tissue plasminogen activator (tPA), which are involved in fibrinolysis and cell migration. This inhibition suggests potential therapeutic applications in managing conditions like thrombosis and cancer progression.
- Cytotoxic Effects : The compound exhibits cytotoxicity against various cancer cell lines by potentially disrupting cellular processes through DNA interaction or enzyme inhibition .
Antimicrobial Activity
Research indicates that 1,4-DCI possesses antimicrobial properties. In vitro studies have demonstrated its effectiveness against several microbial strains, although specific IC50 values and detailed mechanisms remain to be fully elucidated .
Anticancer Activity
1,4-DCI has been evaluated for its anticancer potential through various studies:
- Cell Line Studies : In vitro assays have shown that derivatives of 1,4-DCI can induce cytotoxic effects on cancer cell lines such as HepG2 (liver cancer) and others. The selectivity index (SI), which measures the ratio of cytotoxicity to antiparasitic activity, has been explored to assess the safety profile of these compounds .
- Case Studies : A notable study highlighted the compound's ability to inhibit cancer cell proliferation through mechanisms involving apoptosis induction and cell cycle arrest. For instance, specific derivatives demonstrated enhanced activity compared to the parent compound .
Comparative Analysis with Related Compounds
To better understand the unique properties of 1,4-DCI, a comparison with similar compounds is presented in the following table:
Compound Name | Structure | Key Features |
---|---|---|
1-Chloroisoquinoline | C_9H_7ClN | Contains one chlorine atom; less reactive than 1,4-DCI. |
1,3-Dichloroisoquinoline | C_9H_6Cl_2N | Chlorination at positions 1 and 3; different reactivity profile. |
1-Amino-4-chloroisoquinoline | C_9H_8ClN | Contains an amino group; enhances biological activity compared to dichloro variant. |
6-Chloroisoquinoline | C_9H_6ClN | Chlorination at position 6; different pharmacological properties. |
This comparison illustrates how variations in substitution patterns influence both reactivity and biological activity.
Research Findings
Recent studies have focused on synthesizing new derivatives of 1,4-DCI to enhance its bioactivity:
- Synthesis Techniques : Novel synthetic routes have been developed utilizing readily available starting materials under optimized conditions. Such methods allow for the introduction of various functional groups that can enhance biological efficacy.
- In Vitro Evaluations : Investigations into the antiprotozoal activity against parasites like Plasmodium falciparum have shown promising results for specific derivatives, indicating broader applications beyond traditional antimicrobial and anticancer roles .
Q & A
Basic Research Questions
Q. What are the established synthetic routes for 1,4-Dichloroisoquinoline, and how can reaction parameters be optimized for reproducibility?
- Methodological Answer : The most common synthesis involves heating isocarbostyril with phosphorus oxychloride (POCl₃) in a sealed tube at 120°C for 4 hours, yielding this compound with a melting point of 92–94°C after recrystallization . Alternative methods use phosphorus pentachloride (PCl₅) at 140°C for 6 hours, achieving 50% yield . Optimization includes controlling reaction temperature (±5°C), stoichiometric ratios (excess POCl₃ or PCl₅), and post-reaction purification via steam distillation or ethanol-water recrystallization.
Q. Which spectroscopic and analytical techniques are critical for characterizing this compound?
- Methodological Answer : Nuclear Magnetic Resonance (¹H/¹³C NMR) identifies chlorine substitution patterns via deshielding effects (e.g., aromatic protons adjacent to Cl). Infrared (IR) spectroscopy confirms C-Cl stretches at 550–600 cm⁻¹. High-resolution mass spectrometry (HRMS) validates molecular weight (198.05 g/mol) and isotopic patterns. Elemental analysis (C, H, N) should align with theoretical values (C: 54.58%, H: 2.54%, N: 7.07%) to confirm purity .
Q. What safety protocols are essential when handling this compound in the laboratory?
- Methodological Answer : Refer to GHS hazard codes H315 (skin irritation), H319 (eye irritation), and H335 (respiratory irritation). Use fume hoods, nitrile gloves, and safety goggles. Store under inert atmosphere (argon/nitrogen) at room temperature to prevent decomposition. Spill management involves neutralization with sodium bicarbonate and disposal via hazardous waste protocols .
Advanced Research Questions
Q. How can researchers address discrepancies in reported physicochemical properties (e.g., melting points) of this compound?
- Methodological Answer : Discrepancies (e.g., 88–89°C vs. 92–94°C ) may arise from impurities or polymorphic forms. Resolve via:
- Purity Analysis : HPLC with UV detection (λ = 254 nm) to quantify impurities.
- Recrystallization : Use ethanol-water mixtures to isolate pure crystalline forms.
- Differential Scanning Calorimetry (DSC) : Confirm phase transitions and thermal stability.
Q. What strategies enable selective functionalization of this compound for pharmaceutical applications?
- Methodological Answer : The 1- and 4-chlorine atoms exhibit distinct reactivity:
- Position 1 : Susceptible to nucleophilic aromatic substitution (e.g., with amines or alkoxides) under mild conditions (60–80°C, DMF solvent).
- Position 4 : Requires harsher conditions (Pd-catalyzed cross-coupling, 100–120°C) due to steric hindrance.
Monitor reactions via TLC and characterize intermediates using LC-MS .
Q. How do electronic and steric effects of chlorine substituents influence this compound’s reactivity in catalytic reactions?
- Methodological Answer : Chlorine at position 1 withdraws electron density, activating the ring for electrophilic attacks. Position 4 chlorine creates steric hindrance, limiting access to the heterocyclic core. Computational studies (DFT) predict reactive sites: Fukui indices highlight C-3 and C-8 as electrophilic centers. Validate with kinetic experiments (e.g., Suzuki-Miyaura coupling) .
Q. What mechanistic insights explain the formation of polymeric byproducts during this compound synthesis?
- Methodological Answer : Polymeric byproducts arise from radical intermediates during prolonged heating. Mitigation strategies include:
- Reaction Quenching : Rapid cooling post-reaction to terminate radical chain propagation.
- Additives : Radical scavengers (e.g., TEMPO) suppress polymerization.
- Solvent Choice : Polar aprotic solvents (e.g., DCE) reduce side reactions .
Q. Data Analysis and Experimental Design
Q. How should researchers design experiments to compare the biological activity of this compound derivatives?
- Methodological Answer : Use a standardized panel:
- In vitro assays : Measure IC₅₀ against cancer cell lines (e.g., MCF-7, HeLa) via MTT assay.
- Structure-Activity Relationship (SAR) : Synthesize derivatives with varying substituents (e.g., -Br, -OMe) at positions 1 and 4.
- Statistical Analysis : Apply ANOVA to compare efficacy, with p < 0.05 indicating significance .
Q. What computational tools are effective in predicting the reactivity of this compound in silico?
- Methodological Answer :
- DFT Calculations : Gaussian or ORCA software to compute Fukui indices and molecular electrostatic potentials (MEPs).
- Docking Studies : AutoDock Vina to simulate interactions with biological targets (e.g., kinases).
- QSPR Models : Correlate substituent effects with experimental reaction rates .
Q. How can contradictory data in catalytic applications of this compound be systematically analyzed?
- Methodological Answer : Apply the Cochrane systematic review framework :
- Data Extraction : Tabulate yields, conditions, and catalyst loadings from literature.
- Meta-Analysis : Use RevMan to identify outliers and heterogeneity (I² statistic).
- Sensitivity Testing : Replicate experiments under reported conditions to validate reproducibility.
Properties
IUPAC Name |
1,4-dichloroisoquinoline | |
---|---|---|
Source | PubChem | |
URL | https://pubchem.ncbi.nlm.nih.gov | |
Description | Data deposited in or computed by PubChem | |
InChI |
InChI=1S/C9H5Cl2N/c10-8-5-12-9(11)7-4-2-1-3-6(7)8/h1-5H | |
Source | PubChem | |
URL | https://pubchem.ncbi.nlm.nih.gov | |
Description | Data deposited in or computed by PubChem | |
InChI Key |
HAOKHVBZPIURMZ-UHFFFAOYSA-N | |
Source | PubChem | |
URL | https://pubchem.ncbi.nlm.nih.gov | |
Description | Data deposited in or computed by PubChem | |
Canonical SMILES |
C1=CC=C2C(=C1)C(=CN=C2Cl)Cl | |
Source | PubChem | |
URL | https://pubchem.ncbi.nlm.nih.gov | |
Description | Data deposited in or computed by PubChem | |
Molecular Formula |
C9H5Cl2N | |
Source | PubChem | |
URL | https://pubchem.ncbi.nlm.nih.gov | |
Description | Data deposited in or computed by PubChem | |
DSSTOX Substance ID |
DTXSID30348826 | |
Record name | 1,4-dichloroisoquinoline | |
Source | EPA DSSTox | |
URL | https://comptox.epa.gov/dashboard/DTXSID30348826 | |
Description | DSSTox provides a high quality public chemistry resource for supporting improved predictive toxicology. | |
Molecular Weight |
198.05 g/mol | |
Source | PubChem | |
URL | https://pubchem.ncbi.nlm.nih.gov | |
Description | Data deposited in or computed by PubChem | |
CAS No. |
15298-58-5 | |
Record name | 1,4-dichloroisoquinoline | |
Source | EPA DSSTox | |
URL | https://comptox.epa.gov/dashboard/DTXSID30348826 | |
Description | DSSTox provides a high quality public chemistry resource for supporting improved predictive toxicology. | |
Retrosynthesis Analysis
AI-Powered Synthesis Planning: Our tool employs the Template_relevance Pistachio, Template_relevance Bkms_metabolic, Template_relevance Pistachio_ringbreaker, Template_relevance Reaxys, Template_relevance Reaxys_biocatalysis model, leveraging a vast database of chemical reactions to predict feasible synthetic routes.
One-Step Synthesis Focus: Specifically designed for one-step synthesis, it provides concise and direct routes for your target compounds, streamlining the synthesis process.
Accurate Predictions: Utilizing the extensive PISTACHIO, BKMS_METABOLIC, PISTACHIO_RINGBREAKER, REAXYS, REAXYS_BIOCATALYSIS database, our tool offers high-accuracy predictions, reflecting the latest in chemical research and data.
Strategy Settings
Precursor scoring | Relevance Heuristic |
---|---|
Min. plausibility | 0.01 |
Model | Template_relevance |
Template Set | Pistachio/Bkms_metabolic/Pistachio_ringbreaker/Reaxys/Reaxys_biocatalysis |
Top-N result to add to graph | 6 |
Feasible Synthetic Routes
Disclaimer and Information on In-Vitro Research Products
Please be aware that all articles and product information presented on BenchChem are intended solely for informational purposes. The products available for purchase on BenchChem are specifically designed for in-vitro studies, which are conducted outside of living organisms. In-vitro studies, derived from the Latin term "in glass," involve experiments performed in controlled laboratory settings using cells or tissues. It is important to note that these products are not categorized as medicines or drugs, and they have not received approval from the FDA for the prevention, treatment, or cure of any medical condition, ailment, or disease. We must emphasize that any form of bodily introduction of these products into humans or animals is strictly prohibited by law. It is essential to adhere to these guidelines to ensure compliance with legal and ethical standards in research and experimentation.