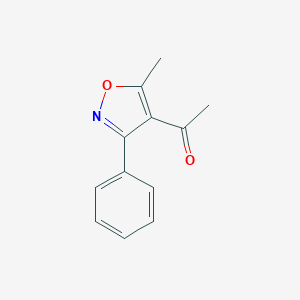
1-(5-Methyl-3-phenylisoxazol-4-yl)ethanone
Overview
Description
“1-(5-Methyl-3-phenylisoxazol-4-yl)ethanone” is a chemical compound with the molecular formula C12H11NO2 . It is synthesized by a 1,3-dipolar cycloaddition reaction of nitrile oxides with sodium pentane-2,4-dionate .
Synthesis Analysis
The synthesis of “1-(5-Methyl-3-phenylisoxazol-4-yl)ethanone” involves a 1,3-dipolar cycloaddition reaction of nitrile oxides with sodium pentane-2,4-dionate . The reaction produces the 5-phenyl and 4-phenyl regioisomers in a 25–30:75–70 ratio . This result is opposite to that obtained utilizing methyl cinnamate as the dipolarophile .
Molecular Structure Analysis
In the molecule, the isoxazole and phenyl rings make a dihedral angle of 84.8 (1)° . The C-C bond distance of the isoxazole molecule present in both DMI and CDMI were found to be 1:432 # A and 1:366 # A, respectively which are in agreement with the experimental values . The C-C bond connecting the isoxazole and methyl group was found to be 1.49 # A .
Scientific Research Applications
Proteomics Research
“1-(5-Methyl-3-phenylisoxazol-4-yl)ethanone” is used as a specialty product for proteomics research .
Synthesis of Derivatives
This compound is used in the synthesis of its derivatives, such as “(3-Phenylisoxazol-5-yl)methanol” derivatives . These derivatives have been synthesized in moderate to excellent yields, indicating the efficiency of the process .
Biological and Pharmaceutical Properties
The “(3-Phenylisoxazol-5-yl)methanol” derivatives synthesized from “1-(5-Methyl-3-phenylisoxazol-4-yl)ethanone” have been found to possess biological and pharmaceutical properties .
Inhibitor of COX-2
A sodium salt derivative of “1-(5-Methyl-3-phenylisoxazol-4-yl)ethanone”, known as “N-[[(5-methyl-3-phenylisoxazol-4-yl)phenyl] sulfonyl]propanamide”, has been identified as a potent and selective inhibitor of COX-2 .
Therapeutic Potential
Isoxazole, which is a family that “1-(5-Methyl-3-phenylisoxazol-4-yl)ethanone” belongs to, has been found to have a wide spectrum of biological activities and therapeutic potential . This includes potential as an analgesic, anti-inflammatory, anticancer, antimicrobial, antiviral, anticonvulsant, antidepressant, and immunosuppressant .
Development of Clinically Viable Drugs
The isoxazole nucleus, which is present in “1-(5-Methyl-3-phenylisoxazol-4-yl)ethanone”, is being studied for the development of clinically viable drugs . This is due to the promising biological activities of isoxazole derivatives .
properties
IUPAC Name |
1-(5-methyl-3-phenyl-1,2-oxazol-4-yl)ethanone | |
---|---|---|
Source | PubChem | |
URL | https://pubchem.ncbi.nlm.nih.gov | |
Description | Data deposited in or computed by PubChem | |
InChI |
InChI=1S/C12H11NO2/c1-8(14)11-9(2)15-13-12(11)10-6-4-3-5-7-10/h3-7H,1-2H3 | |
Source | PubChem | |
URL | https://pubchem.ncbi.nlm.nih.gov | |
Description | Data deposited in or computed by PubChem | |
InChI Key |
LWPHDEBMXBDBBO-UHFFFAOYSA-N | |
Source | PubChem | |
URL | https://pubchem.ncbi.nlm.nih.gov | |
Description | Data deposited in or computed by PubChem | |
Canonical SMILES |
CC1=C(C(=NO1)C2=CC=CC=C2)C(=O)C | |
Source | PubChem | |
URL | https://pubchem.ncbi.nlm.nih.gov | |
Description | Data deposited in or computed by PubChem | |
Molecular Formula |
C12H11NO2 | |
Source | PubChem | |
URL | https://pubchem.ncbi.nlm.nih.gov | |
Description | Data deposited in or computed by PubChem | |
DSSTOX Substance ID |
DTXSID20312475 | |
Record name | 1-(5-Methyl-3-phenyl-1,2-oxazol-4-yl)ethan-1-one | |
Source | EPA DSSTox | |
URL | https://comptox.epa.gov/dashboard/DTXSID20312475 | |
Description | DSSTox provides a high quality public chemistry resource for supporting improved predictive toxicology. | |
Molecular Weight |
201.22 g/mol | |
Source | PubChem | |
URL | https://pubchem.ncbi.nlm.nih.gov | |
Description | Data deposited in or computed by PubChem | |
Product Name |
1-(5-Methyl-3-phenylisoxazol-4-yl)ethanone | |
CAS RN |
19212-42-1 | |
Record name | 19212-42-1 | |
Source | DTP/NCI | |
URL | https://dtp.cancer.gov/dtpstandard/servlet/dwindex?searchtype=NSC&outputformat=html&searchlist=255230 | |
Description | The NCI Development Therapeutics Program (DTP) provides services and resources to the academic and private-sector research communities worldwide to facilitate the discovery and development of new cancer therapeutic agents. | |
Explanation | Unless otherwise indicated, all text within NCI products is free of copyright and may be reused without our permission. Credit the National Cancer Institute as the source. | |
Record name | 1-(5-Methyl-3-phenyl-1,2-oxazol-4-yl)ethan-1-one | |
Source | EPA DSSTox | |
URL | https://comptox.epa.gov/dashboard/DTXSID20312475 | |
Description | DSSTox provides a high quality public chemistry resource for supporting improved predictive toxicology. | |
Synthesis routes and methods
Procedure details
Q & A
Q1: What is the molecular structure of 1-(5-Methyl-3-phenylisoxazol-4-yl)ethanone and how was it synthesized?
A1: 1-(5-Methyl-3-phenylisoxazol-4-yl)ethanone is an organic compound featuring an isoxazole ring core. [] It was synthesized through a 1,3-dipolar cycloaddition reaction. This type of reaction involves a nitrile oxide reacting with sodium pentane-2,4-dionate to form the isoxazole ring structure. [] A key structural feature of this compound is the dihedral angle of 84.8° between the isoxazole and phenyl rings within the molecule. []
Disclaimer and Information on In-Vitro Research Products
Please be aware that all articles and product information presented on BenchChem are intended solely for informational purposes. The products available for purchase on BenchChem are specifically designed for in-vitro studies, which are conducted outside of living organisms. In-vitro studies, derived from the Latin term "in glass," involve experiments performed in controlled laboratory settings using cells or tissues. It is important to note that these products are not categorized as medicines or drugs, and they have not received approval from the FDA for the prevention, treatment, or cure of any medical condition, ailment, or disease. We must emphasize that any form of bodily introduction of these products into humans or animals is strictly prohibited by law. It is essential to adhere to these guidelines to ensure compliance with legal and ethical standards in research and experimentation.