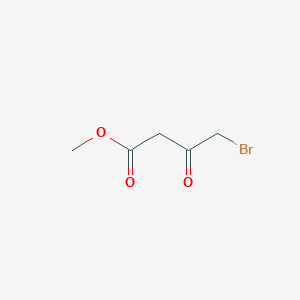
Methyl 4-bromo-3-oxobutanoate
Overview
Description
Methyl 4-bromo-3-oxobutanoate is an organic compound with the molecular formula C5H7BrO3. It is a brominated ester that is commonly used in organic synthesis due to its reactivity and versatility. The compound is characterized by the presence of a bromine atom attached to the fourth carbon of a butanoate ester, which also contains a ketone group at the third carbon position.
Preparation Methods
Synthetic Routes and Reaction Conditions: Methyl 4-bromo-3-oxobutanoate can be synthesized through several methods. One common approach involves the bromination of methyl acetoacetate. The reaction typically uses bromine (Br2) as the brominating agent in the presence of a solvent such as acetic acid or carbon tetrachloride. The reaction proceeds under mild conditions, often at room temperature, to yield this compound.
Industrial Production Methods: In an industrial setting, the production of this compound may involve continuous flow processes to ensure high yield and purity. The use of automated systems allows for precise control of reaction parameters such as temperature, concentration, and reaction time. This ensures consistent production quality and scalability.
Chemical Reactions Analysis
Types of Reactions: Methyl 4-bromo-3-oxobutanoate undergoes various chemical reactions, including:
Nucleophilic Substitution: The bromine atom can be replaced by other nucleophiles such as amines, thiols, or alkoxides.
Reduction: The ketone group can be reduced to a hydroxyl group using reducing agents like sodium borohydride (NaBH4) or lithium aluminum hydride (LiAlH4).
Condensation Reactions: The compound can participate in aldol condensations, forming larger carbon frameworks.
Common Reagents and Conditions:
Nucleophilic Substitution: Reagents such as sodium azide (NaN3) or potassium thiocyanate (KSCN) in polar aprotic solvents like dimethylformamide (DMF).
Reduction: Sodium borohydride (NaBH4) in methanol or lithium aluminum hydride (LiAlH4) in ether.
Condensation: Base catalysts like sodium hydroxide (NaOH) or potassium hydroxide (KOH) in aqueous or alcoholic solutions.
Major Products:
Nucleophilic Substitution: Products include azides, thiocyanates, and ethers.
Reduction: The major product is methyl 4-bromo-3-hydroxybutanoate.
Condensation: Products include β-hydroxy esters and α,β-unsaturated esters.
Scientific Research Applications
Methyl 4-bromo-3-oxobutanoate is widely used in scientific research due to its reactivity and versatility. Some of its applications include:
Organic Synthesis: It serves as a building block for the synthesis of various complex organic molecules, including pharmaceuticals and agrochemicals.
Medicinal Chemistry: The compound is used in the synthesis of biologically active molecules, including potential drug candidates.
Material Science: It is used in the preparation of polymers and advanced materials with specific properties.
Biological Studies: The compound is used in the synthesis of enzyme inhibitors and other biologically relevant molecules.
Mechanism of Action
The mechanism of action of methyl 4-bromo-3-oxobutanoate in chemical reactions involves the reactivity of the bromine atom and the ketone group. The bromine atom is a good leaving group, making the compound susceptible to nucleophilic substitution reactions. The ketone group can undergo reduction and condensation reactions due to the electrophilic nature of the carbonyl carbon.
Molecular Targets and Pathways: In biological systems, the compound may interact with enzymes and proteins through covalent modification or inhibition. The specific molecular targets and pathways depend on the structure of the final synthesized molecules derived from this compound.
Comparison with Similar Compounds
Methyl 4-bromo-3-oxobutanoate can be compared with other similar compounds such as:
Ethyl 4-bromo-3-oxobutanoate: Similar in structure but with an ethyl ester group instead of a methyl ester group.
Methyl 4-chloro-3-oxobutanoate: Similar in structure but with a chlorine atom instead of a bromine atom.
Methyl 3-oxobutanoate: Lacks the bromine atom, making it less reactive in nucleophilic substitution reactions.
Uniqueness: The presence of the bromine atom in this compound makes it more reactive in nucleophilic substitution reactions compared to its chloro and non-halogenated counterparts. This reactivity is advantageous in synthetic applications where selective substitution is required.
Properties
IUPAC Name |
methyl 4-bromo-3-oxobutanoate | |
---|---|---|
Source | PubChem | |
URL | https://pubchem.ncbi.nlm.nih.gov | |
Description | Data deposited in or computed by PubChem | |
InChI |
InChI=1S/C5H7BrO3/c1-9-5(8)2-4(7)3-6/h2-3H2,1H3 | |
Source | PubChem | |
URL | https://pubchem.ncbi.nlm.nih.gov | |
Description | Data deposited in or computed by PubChem | |
InChI Key |
CZRWOPRGDPUSDE-UHFFFAOYSA-N | |
Source | PubChem | |
URL | https://pubchem.ncbi.nlm.nih.gov | |
Description | Data deposited in or computed by PubChem | |
Canonical SMILES |
COC(=O)CC(=O)CBr | |
Source | PubChem | |
URL | https://pubchem.ncbi.nlm.nih.gov | |
Description | Data deposited in or computed by PubChem | |
Molecular Formula |
C5H7BrO3 | |
Source | PubChem | |
URL | https://pubchem.ncbi.nlm.nih.gov | |
Description | Data deposited in or computed by PubChem | |
DSSTOX Substance ID |
DTXSID00304277 | |
Record name | Methyl 4-bromo-3-oxobutanoate | |
Source | EPA DSSTox | |
URL | https://comptox.epa.gov/dashboard/DTXSID00304277 | |
Description | DSSTox provides a high quality public chemistry resource for supporting improved predictive toxicology. | |
Molecular Weight |
195.01 g/mol | |
Source | PubChem | |
URL | https://pubchem.ncbi.nlm.nih.gov | |
Description | Data deposited in or computed by PubChem | |
CAS No. |
17790-81-7 | |
Record name | NSC165240 | |
Source | DTP/NCI | |
URL | https://dtp.cancer.gov/dtpstandard/servlet/dwindex?searchtype=NSC&outputformat=html&searchlist=165240 | |
Description | The NCI Development Therapeutics Program (DTP) provides services and resources to the academic and private-sector research communities worldwide to facilitate the discovery and development of new cancer therapeutic agents. | |
Explanation | Unless otherwise indicated, all text within NCI products is free of copyright and may be reused without our permission. Credit the National Cancer Institute as the source. | |
Record name | Methyl 4-bromo-3-oxobutanoate | |
Source | EPA DSSTox | |
URL | https://comptox.epa.gov/dashboard/DTXSID00304277 | |
Description | DSSTox provides a high quality public chemistry resource for supporting improved predictive toxicology. | |
Synthesis routes and methods I
Procedure details
Synthesis routes and methods II
Procedure details
Synthesis routes and methods III
Procedure details
Synthesis routes and methods IV
Procedure details
Retrosynthesis Analysis
AI-Powered Synthesis Planning: Our tool employs the Template_relevance Pistachio, Template_relevance Bkms_metabolic, Template_relevance Pistachio_ringbreaker, Template_relevance Reaxys, Template_relevance Reaxys_biocatalysis model, leveraging a vast database of chemical reactions to predict feasible synthetic routes.
One-Step Synthesis Focus: Specifically designed for one-step synthesis, it provides concise and direct routes for your target compounds, streamlining the synthesis process.
Accurate Predictions: Utilizing the extensive PISTACHIO, BKMS_METABOLIC, PISTACHIO_RINGBREAKER, REAXYS, REAXYS_BIOCATALYSIS database, our tool offers high-accuracy predictions, reflecting the latest in chemical research and data.
Strategy Settings
Precursor scoring | Relevance Heuristic |
---|---|
Min. plausibility | 0.01 |
Model | Template_relevance |
Template Set | Pistachio/Bkms_metabolic/Pistachio_ringbreaker/Reaxys/Reaxys_biocatalysis |
Top-N result to add to graph | 6 |
Feasible Synthetic Routes
Disclaimer and Information on In-Vitro Research Products
Please be aware that all articles and product information presented on BenchChem are intended solely for informational purposes. The products available for purchase on BenchChem are specifically designed for in-vitro studies, which are conducted outside of living organisms. In-vitro studies, derived from the Latin term "in glass," involve experiments performed in controlled laboratory settings using cells or tissues. It is important to note that these products are not categorized as medicines or drugs, and they have not received approval from the FDA for the prevention, treatment, or cure of any medical condition, ailment, or disease. We must emphasize that any form of bodily introduction of these products into humans or animals is strictly prohibited by law. It is essential to adhere to these guidelines to ensure compliance with legal and ethical standards in research and experimentation.