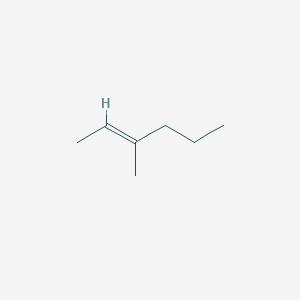
3-Methyl-2-hexene
Overview
Description
3-Methyl-2-hexene is an organic compound with the molecular formula C7H14 . It contains a total of 21 atoms, including 14 Hydrogen atoms and 7 Carbon atoms .
Synthesis Analysis
The synthesis of 3-Methyl-2-hexene can be achieved through multiple step pathways. For instance, one approach could be to reduce the alkyne to cis or trans-3-hexene before undertaking glycol formation . Another method involves the use of ethyl bromide and 2-pentanone .Molecular Structure Analysis
The molecular structure of 3-Methyl-2-hexene includes the arrangement of atoms and the chemical bonds that hold the atoms together. It contains a total of 20 bonds, including 6 non-H bonds, 1 multiple bond, 2 rotatable bonds, and 1 double bond .Physical And Chemical Properties Analysis
The physical and chemical properties of 3-Methyl-2-hexene include its molecular weight of 98.1861 . More detailed thermophysical property data can be found in the NIST/TRC Web Thermo Tables .Scientific Research Applications
1. Dimerization and Isomerization in Chemical Reactions
Research on 3-Methyl-2-hexene has shown its involvement in dimerization and isomerization processes. For instance, the dimerization of propylene over a nickel oxide-silica-alumina catalyst leads to the production of several hexene isomers, including 3-Methyl-2-hexene (Imai, Hasegawa, & Uchida, 1968). Similarly, the catalytic isomerization of 1-hexene on H-ZSM-5 zeolite has shown that 3-Methyl-2-hexene can be a product of such reactions, highlighting the influence of catalyst pore structure (Abbot, Corma, & Wojciechowski, 1985).
2. Potential in Fuel Synthesis
The compound has been explored in the context of renewable fuel synthesis. Research demonstrates the possibility of using isomers like 3-Methyl-2-hexene in the synthesis of jet and diesel fuels. For example, the dimerization of 2-ethyl-1-hexene to a mixture of hydrocarbons, including 3-Methyl-2-hexene isomers, suggests potential applications in creating renewable fuels (Harvey & Quintana, 2010).
3. Role in Polymerization and Chemical Structures
Studies on polymerization show that 3-Methyl-2-hexene and its isomers can be involved in the formation of various polymers. For instance, the polymerization of different α-olefins, including 5-methyl-1-hexene, demonstrates the creation of highly crystalline polymers, suggesting the potential role of 3-Methyl-2-hexene in similar processes (Natta et al., 1967).
4. Applications in Catalysis and Oxidation Processes
The compound's involvement in various catalytic and oxidation processes has been documented. Studies on the catalytic activity of silica gel in isomerizing 1-hexene, which produces various hexene isomers including 3-Methyl-2-hexene, shed light on its potential applications in catalysis (West, 1973). Additionally, the oxidation of 1-hexene in various solvents and the selective production of 2-hexanone indicate the potential utility of 3-Methyl-2-hexene in oxidation reactions (Hou et al., 2002).
Safety and Hazards
Safety measures for handling 3-Methyl-2-hexene include avoiding dust formation, breathing mist, gas or vapors, and contact with skin and eye. It is recommended to use personal protective equipment, ensure adequate ventilation, remove all sources of ignition, and evacuate personnel to safe areas . It’s also important to note that vapors may form explosive mixtures with air .
properties
IUPAC Name |
(E)-3-methylhex-2-ene | |
---|---|---|
Source | PubChem | |
URL | https://pubchem.ncbi.nlm.nih.gov | |
Description | Data deposited in or computed by PubChem | |
InChI |
InChI=1S/C7H14/c1-4-6-7(3)5-2/h5H,4,6H2,1-3H3/b7-5+ | |
Source | PubChem | |
URL | https://pubchem.ncbi.nlm.nih.gov | |
Description | Data deposited in or computed by PubChem | |
InChI Key |
JZMUUSXQSKCZNO-FNORWQNLSA-N | |
Source | PubChem | |
URL | https://pubchem.ncbi.nlm.nih.gov | |
Description | Data deposited in or computed by PubChem | |
Canonical SMILES |
CCCC(=CC)C | |
Source | PubChem | |
URL | https://pubchem.ncbi.nlm.nih.gov | |
Description | Data deposited in or computed by PubChem | |
Isomeric SMILES |
CCC/C(=C/C)/C | |
Source | PubChem | |
URL | https://pubchem.ncbi.nlm.nih.gov | |
Description | Data deposited in or computed by PubChem | |
Molecular Formula |
C7H14 | |
Source | PubChem | |
URL | https://pubchem.ncbi.nlm.nih.gov | |
Description | Data deposited in or computed by PubChem | |
DSSTOX Substance ID |
DTXSID701025650 | |
Record name | (E)-3-Methyl-2-hexene | |
Source | EPA DSSTox | |
URL | https://comptox.epa.gov/dashboard/DTXSID701025650 | |
Description | DSSTox provides a high quality public chemistry resource for supporting improved predictive toxicology. | |
Molecular Weight |
98.19 g/mol | |
Source | PubChem | |
URL | https://pubchem.ncbi.nlm.nih.gov | |
Description | Data deposited in or computed by PubChem | |
CAS RN |
20710-38-7, 17618-77-8 | |
Record name | (2E)-3-Methyl-2-hexene | |
Source | CAS Common Chemistry | |
URL | https://commonchemistry.cas.org/detail?cas_rn=20710-38-7 | |
Description | CAS Common Chemistry is an open community resource for accessing chemical information. Nearly 500,000 chemical substances from CAS REGISTRY cover areas of community interest, including common and frequently regulated chemicals, and those relevant to high school and undergraduate chemistry classes. This chemical information, curated by our expert scientists, is provided in alignment with our mission as a division of the American Chemical Society. | |
Explanation | The data from CAS Common Chemistry is provided under a CC-BY-NC 4.0 license, unless otherwise stated. | |
Record name | 3-Methyl-2-hexene | |
Source | ChemIDplus | |
URL | https://pubchem.ncbi.nlm.nih.gov/substance/?source=chemidplus&sourceid=0017618778 | |
Description | ChemIDplus is a free, web search system that provides access to the structure and nomenclature authority files used for the identification of chemical substances cited in National Library of Medicine (NLM) databases, including the TOXNET system. | |
Record name | (E)-3-Methyl-2-hexene | |
Source | ChemIDplus | |
URL | https://pubchem.ncbi.nlm.nih.gov/substance/?source=chemidplus&sourceid=0020710387 | |
Description | ChemIDplus is a free, web search system that provides access to the structure and nomenclature authority files used for the identification of chemical substances cited in National Library of Medicine (NLM) databases, including the TOXNET system. | |
Record name | (E)-3-Methyl-2-hexene | |
Source | DTP/NCI | |
URL | https://dtp.cancer.gov/dtpstandard/servlet/dwindex?searchtype=NSC&outputformat=html&searchlist=73931 | |
Description | The NCI Development Therapeutics Program (DTP) provides services and resources to the academic and private-sector research communities worldwide to facilitate the discovery and development of new cancer therapeutic agents. | |
Explanation | Unless otherwise indicated, all text within NCI products is free of copyright and may be reused without our permission. Credit the National Cancer Institute as the source. | |
Record name | (E)-3-Methyl-2-hexene | |
Source | EPA DSSTox | |
URL | https://comptox.epa.gov/dashboard/DTXSID701025650 | |
Description | DSSTox provides a high quality public chemistry resource for supporting improved predictive toxicology. | |
Record name | 3-Methyl-2-hexene (cis- and trans- mixture) | |
Source | European Chemicals Agency (ECHA) | |
URL | https://echa.europa.eu/information-on-chemicals | |
Description | The European Chemicals Agency (ECHA) is an agency of the European Union which is the driving force among regulatory authorities in implementing the EU's groundbreaking chemicals legislation for the benefit of human health and the environment as well as for innovation and competitiveness. | |
Explanation | Use of the information, documents and data from the ECHA website is subject to the terms and conditions of this Legal Notice, and subject to other binding limitations provided for under applicable law, the information, documents and data made available on the ECHA website may be reproduced, distributed and/or used, totally or in part, for non-commercial purposes provided that ECHA is acknowledged as the source: "Source: European Chemicals Agency, http://echa.europa.eu/". Such acknowledgement must be included in each copy of the material. ECHA permits and encourages organisations and individuals to create links to the ECHA website under the following cumulative conditions: Links can only be made to webpages that provide a link to the Legal Notice page. | |
Retrosynthesis Analysis
AI-Powered Synthesis Planning: Our tool employs the Template_relevance Pistachio, Template_relevance Bkms_metabolic, Template_relevance Pistachio_ringbreaker, Template_relevance Reaxys, Template_relevance Reaxys_biocatalysis model, leveraging a vast database of chemical reactions to predict feasible synthetic routes.
One-Step Synthesis Focus: Specifically designed for one-step synthesis, it provides concise and direct routes for your target compounds, streamlining the synthesis process.
Accurate Predictions: Utilizing the extensive PISTACHIO, BKMS_METABOLIC, PISTACHIO_RINGBREAKER, REAXYS, REAXYS_BIOCATALYSIS database, our tool offers high-accuracy predictions, reflecting the latest in chemical research and data.
Strategy Settings
Precursor scoring | Relevance Heuristic |
---|---|
Min. plausibility | 0.01 |
Model | Template_relevance |
Template Set | Pistachio/Bkms_metabolic/Pistachio_ringbreaker/Reaxys/Reaxys_biocatalysis |
Top-N result to add to graph | 6 |
Feasible Synthetic Routes
Disclaimer and Information on In-Vitro Research Products
Please be aware that all articles and product information presented on BenchChem are intended solely for informational purposes. The products available for purchase on BenchChem are specifically designed for in-vitro studies, which are conducted outside of living organisms. In-vitro studies, derived from the Latin term "in glass," involve experiments performed in controlled laboratory settings using cells or tissues. It is important to note that these products are not categorized as medicines or drugs, and they have not received approval from the FDA for the prevention, treatment, or cure of any medical condition, ailment, or disease. We must emphasize that any form of bodily introduction of these products into humans or animals is strictly prohibited by law. It is essential to adhere to these guidelines to ensure compliance with legal and ethical standards in research and experimentation.