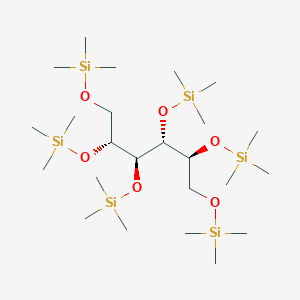
Trimethylsilyldulcitol
- Click on QUICK INQUIRY to receive a quote from our team of experts.
- With the quality product at a COMPETITIVE price, you can focus more on your research.
Overview
Description
Trimethylsilyldulcitol is a derivative of dulcitol, a sugar alcohol. It is characterized by the presence of six trimethylsilyl groups attached to the hydroxyl groups of dulcitol. The empirical formula of this compound is C24H62O6Si6, and it has a molecular weight of 615.26 g/mol . This compound is primarily used in research settings and is not intended for diagnostic or therapeutic use .
Preparation Methods
Synthetic Routes and Reaction Conditions: Trimethylsilyldulcitol can be synthesized through the silylation of dulcitol. The process involves the reaction of dulcitol with trimethylsilyl chloride in the presence of a base such as pyridine or imidazole. The reaction is typically carried out in an anhydrous solvent like dichloromethane or tetrahydrofuran at room temperature.
Industrial Production Methods: While there is limited information on the large-scale industrial production of this compound, the synthesis method mentioned above can be adapted for larger-scale production by optimizing reaction conditions and using appropriate scaling techniques.
Chemical Reactions Analysis
Types of Reactions: Trimethylsilyldulcitol primarily undergoes substitution reactions due to the presence of trimethylsilyl groups. These groups can be replaced by other functional groups under suitable conditions .
Common Reagents and Conditions:
Substitution Reactions: Trimethylsilyl groups can be replaced by other groups using reagents like tetrabutylammonium fluoride (TBAF) in tetrahydrofuran.
Oxidation and Reduction:
Major Products Formed: The major products formed from the substitution reactions of this compound are typically the corresponding hydroxyl derivatives, where the trimethylsilyl groups are replaced by hydroxyl groups .
Scientific Research Applications
Trimethylsilyldulcitol has several applications in scientific research:
Mechanism of Action
The mechanism of action of Trimethylsilyldulcitol involves the interaction of its trimethylsilyl groups with various molecular targets. These interactions can lead to the formation of stable complexes or the substitution of trimethylsilyl groups with other functional groups . The compound’s effects are primarily mediated through its ability to protect hydroxyl groups and facilitate specific chemical reactions .
Comparison with Similar Compounds
Trimethylsilyl chloride: Used in similar silylation reactions but lacks the sugar alcohol backbone of Trimethylsilyldulcitol.
Trimethylsilyl cyanide: Another silylating agent used in organic synthesis.
Hexamethyldisilazane: Used for the protection of hydroxyl groups in organic synthesis.
Uniqueness: this compound is unique due to its combination of a sugar alcohol backbone with multiple trimethylsilyl groups. This structure provides it with distinct properties, making it particularly useful in carbohydrate chemistry and the protection of hydroxyl groups during synthesis .
Properties
IUPAC Name |
trimethyl-[(2S,3R,4S,5R)-1,2,4,5,6-pentakis(trimethylsilyloxy)hexan-3-yl]oxysilane |
Source
|
---|---|---|
Source | PubChem | |
URL | https://pubchem.ncbi.nlm.nih.gov | |
Description | Data deposited in or computed by PubChem | |
InChI |
InChI=1S/C24H62O6Si6/c1-31(2,3)25-19-21(27-33(7,8)9)23(29-35(13,14)15)24(30-36(16,17)18)22(28-34(10,11)12)20-26-32(4,5)6/h21-24H,19-20H2,1-18H3/t21-,22+,23+,24- |
Source
|
Source | PubChem | |
URL | https://pubchem.ncbi.nlm.nih.gov | |
Description | Data deposited in or computed by PubChem | |
InChI Key |
USBJDBWAPKNPCK-NVPYSNMXSA-N |
Source
|
Source | PubChem | |
URL | https://pubchem.ncbi.nlm.nih.gov | |
Description | Data deposited in or computed by PubChem | |
Canonical SMILES |
C[Si](C)(C)OCC(C(C(C(CO[Si](C)(C)C)O[Si](C)(C)C)O[Si](C)(C)C)O[Si](C)(C)C)O[Si](C)(C)C |
Source
|
Source | PubChem | |
URL | https://pubchem.ncbi.nlm.nih.gov | |
Description | Data deposited in or computed by PubChem | |
Isomeric SMILES |
C[Si](C)(C)OC[C@H]([C@@H]([C@@H]([C@H](CO[Si](C)(C)C)O[Si](C)(C)C)O[Si](C)(C)C)O[Si](C)(C)C)O[Si](C)(C)C |
Source
|
Source | PubChem | |
URL | https://pubchem.ncbi.nlm.nih.gov | |
Description | Data deposited in or computed by PubChem | |
Molecular Formula |
C24H62O6Si6 |
Source
|
Source | PubChem | |
URL | https://pubchem.ncbi.nlm.nih.gov | |
Description | Data deposited in or computed by PubChem | |
DSSTOX Substance ID |
DTXSID40550675 |
Source
|
Record name | 1,2,3,4,5,6-Hexakis-O-(trimethylsilyl)-D-galactitol | |
Source | EPA DSSTox | |
URL | https://comptox.epa.gov/dashboard/DTXSID40550675 | |
Description | DSSTox provides a high quality public chemistry resource for supporting improved predictive toxicology. | |
Molecular Weight |
615.3 g/mol |
Source
|
Source | PubChem | |
URL | https://pubchem.ncbi.nlm.nih.gov | |
Description | Data deposited in or computed by PubChem | |
CAS No. |
18919-39-6 |
Source
|
Record name | 1,2,3,4,5,6-Hexakis-O-(trimethylsilyl)-D-galactitol | |
Source | EPA DSSTox | |
URL | https://comptox.epa.gov/dashboard/DTXSID40550675 | |
Description | DSSTox provides a high quality public chemistry resource for supporting improved predictive toxicology. | |
Disclaimer and Information on In-Vitro Research Products
Please be aware that all articles and product information presented on BenchChem are intended solely for informational purposes. The products available for purchase on BenchChem are specifically designed for in-vitro studies, which are conducted outside of living organisms. In-vitro studies, derived from the Latin term "in glass," involve experiments performed in controlled laboratory settings using cells or tissues. It is important to note that these products are not categorized as medicines or drugs, and they have not received approval from the FDA for the prevention, treatment, or cure of any medical condition, ailment, or disease. We must emphasize that any form of bodily introduction of these products into humans or animals is strictly prohibited by law. It is essential to adhere to these guidelines to ensure compliance with legal and ethical standards in research and experimentation.