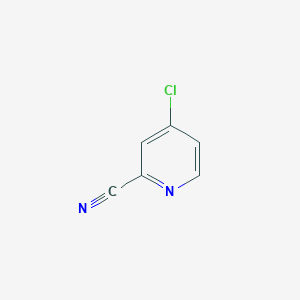
4-Chloropyridine-2-carbonitrile
Overview
Description
4-Chloropyridine-2-carbonitrile (CAS: 19235-89-3) is a heteroaromatic compound with the molecular formula C₆H₃ClN₂. It features a pyridine ring substituted with a chlorine atom at the 4-position and a nitrile group at the 2-position. This compound serves as a versatile intermediate in pharmaceutical synthesis, particularly for preparing heteroaromatic carboxylic acids and substituted pyridinyl nitriles with biological activity . Its physicochemical properties include a melting point of 81–85°C, boiling point of 231.6±20.0°C (760 mmHg), and molecular weight of 138.55 g/mol .
Preparation Methods
One-Step Synthesis from 4-Nitropyridine N-Oxide
A streamlined one-step protocol was developed by Veerareddy et al. (2011), bypassing the need for pre-synthesized N-oxide intermediates. This method reacts 4-nitropyridine N-oxide with ethyl chloroformate and TMSCN in a single pot, achieving simultaneous nitro group reduction and cyanation . The reaction proceeds via in situ generation of a reactive carbamate intermediate, which undergoes nucleophilic displacement by TMSCN.
Key advantages include reduced purification steps and compatibility with diverse N-oxide substrates. However, the yield remains unspecified in published literature, and scalability is limited by the cost of ethyl chloroformate. Temperature control is critical, as excess heat may lead to byproducts such as 4-cyanopyridine derivatives .
Decarboxylative Cyanation from Pyridine-2-Carboxylic Acid
Chlorination and Salt Formation
The industrial synthesis of this compound often begins with pyridine-2-carboxylic acid. In the first step, chlorination is achieved using thionyl chloride (SOCl₂) in the presence of sodium bromide (NaBr) as a catalyst. This step proceeds via electrophilic aromatic substitution, with NaBr enhancing the electrophilicity of SOCl₂ by generating bromine intermediates . Optimal conditions involve refluxing at 80–90°C for 4–6 hours, achieving near-quantitative conversion to 4-chloropyridine-2-carboxylic acid .
The resulting acid is then neutralized with a base such as potassium tert-butoxide (KOtBu) to form a water-soluble salt, facilitating purification. Patent data indicates a molar ratio of 1:1.2 (acid-to-base) in ethanol, yielding 4-chloropyridine-2-carboxylate with >95% purity .
Decarboxylation and Cyanation
The carboxylate salt undergoes decarboxylative cyanation using a copper catalyst (e.g., CuI), potassium cyanide (KCN), and an oxidizing agent (e.g., tert-butyl hydroperoxide) in DMF at 100–120°C . This step leverages radical intermediates to replace the carboxylate group with a nitrile, achieving an overall yield of 78–85% across three steps . Industrial scalability is enhanced by the low cost of starting materials and tolerance to atmospheric moisture.
Trifluoroacetic Anhydride-Mediated Dehydration
A high-yielding laboratory method involves dehydrating 4-chloropyridine-2-carboxamide using trifluoroacetic anhydride (TFAA) and triethylamine (Et₃N). The reaction, conducted in ethyl acetate at −5°C to 20°C, achieves a 90% yield within 1.25 hours . The mechanism proceeds via activation of the amide oxygen by TFAA, followed by elimination of water and trifluoroacetate.
This method is favored for its rapid kinetics and minimal byproducts. However, the requirement for anhydrous conditions and the high cost of TFAA limit its industrial use.
Comparative Analysis of Synthetic Methods
Method | Starting Material | Key Reagents | Conditions | Yield | Scalability |
---|---|---|---|---|---|
Cyanation of N-Oxide | 4-Chloropyridine N-oxide | TMSCN | Reflux, DCM | Not reported | Laboratory-scale |
One-Step from Nitro N-Oxide | 4-Nitropyridine N-oxide | Ethyl chloroformate, TMSCN | Room temperature | Not reported | Small-scale |
Decarboxylative Cyanation | Pyridine-2-carboxylic acid | SOCl₂, NaBr, KCN, CuI | Multi-step, 80–120°C | 78–85% | Industrial |
TFAA Dehydration | 4-Chloropyridine-2-carboxamide | TFAA, Et₃N | −5°C to 20°C, EtOAc | 90% | Laboratory-scale |
Catalytic Bromine-Assisted Chlorination
A notable advancement in the chlorination step (Method 3.1) involves using bromine as a catalyst to enhance regioselectivity. As detailed in JP2011153081A, bromine generates Br⁺ ions that polarize the pyridine ring, directing chlorination to the C-4 position with >99% selectivity . This modification reduces side products like 2,4-dichloropyridine derivatives, which are common in uncatalyzed reactions.
Chemical Reactions Analysis
Types of Reactions: 4-Chloropyridine-2-carbonitrile undergoes various chemical reactions, including:
Nucleophilic Substitution: The chloro substituent can be replaced by strong nucleophiles, leading to the formation of highly functionalized pyridine derivatives.
Cyclization Reactions: The nitrile group can participate in cyclization reactions with amino alcohols in the presence of zinc chloride, forming oxazoline derivatives.
Common Reagents and Conditions:
Nucleophilic Substitution: Strong nucleophiles such as sodium methoxide or ethylenediamine are commonly used.
Cyclization Reactions: Zinc chloride is often employed as a catalyst.
Major Products:
Nucleophilic Substitution: Functionalized pyridine derivatives.
Cyclization Reactions: Oxazoline derivatives with dual coordination capabilities.
Scientific Research Applications
4-Chloropyridine-2-carbonitrile is widely used in scientific research due to its versatility:
Chemistry: It serves as an intermediate in the synthesis of various organic compounds and ligands.
Biology: It is used in the modification of bioactive molecules, enhancing their properties.
Medicine: It plays a role in the development of pharmaceutical intermediates.
Industry: It is utilized in the production of agrochemicals and other industrial chemicals.
Mechanism of Action
The mechanism of action of 4-Chloropyridine-2-carbonitrile involves its ability to participate in nucleophilic substitution and cyclization reactions. The chloro substituent and nitrile group are key functional groups that facilitate these reactions, allowing the compound to interact with various molecular targets and pathways .
Comparison with Similar Compounds
Comparison with Structurally Similar Compounds
Structural Isomers and Positional Analogues
6-Chloropyridine-2-carbonitrile
- Structural Differences : The chlorine atom is positioned at the 6-position instead of the 4-position.
- Intermolecular Interactions : Unlike 4-chloropyridine-2-carbonitrile, which forms 1D chains via head-to-head C–H⋯N interactions, 6-chloropyridine-2-carbonitrile adopts a 2D sheet structure through head-to-tail interactions. Both exhibit π-stacking, but the offset distances differ (4.11 Å for 4-chloro vs. 3.90 Å for 6-chloro) .
- Synthetic Routes : Both isomers can be synthesized via cyanation of pyridine N-oxides using trimethylsilanecarbonitrile (TMSCN) .
2-Chloropyridine-4-carbonitrile (LOBVIJ)
- Key Features : The chlorine and nitrile groups are transposed (Cl at 2-position, CN at 4-position).
- Bond Metrics : The nitrile bond length (1.141 Å) is nearly identical to those in this compound (1.156 Å) and 6-chloropyridine-2-carbonitrile (1.138 Å) .
Derivatives with Additional Functional Groups
4-Chloropyridine-2,6-dicarbonitrile (CAS: 55306-66-6)
- Structure : Contains two nitrile groups at positions 2 and 6, with chlorine at position 3.
- Applications: Used in coordination chemistry and as a ligand precursor. Its higher polarity (compared to mono-nitrile analogues) influences solubility and reactivity .
6-(Chloromethyl)-2-cyanopyridine (CAS: 135450-23-6)
Physicochemical Properties Comparison
Compound | Molecular Weight (g/mol) | Melting Point (°C) | Boiling Point (°C) | Nitrile Bond Length (Å) |
---|---|---|---|---|
This compound | 138.55 | 81–85 | 231.6 ± 20.0 | 1.156 |
6-Chloropyridine-2-carbonitrile | 138.55 | Not reported | Not reported | 1.138 |
2-Chloropyridine-4-carbonitrile | 138.55 | Not reported | Not reported | 1.141 |
4-Chloropyridine-2,6-dicarbonitrile | 163.56 | Not reported | Not reported | ~1.14 (avg.) |
Data sourced from crystallographic studies and commercial specifications .
Biological Activity
4-Chloropyridine-2-carbonitrile is an organic compound that has garnered attention in pharmaceutical chemistry due to its diverse biological activities. This article provides a comprehensive overview of its synthesis, biological properties, and potential applications based on recent research findings.
Chemical Structure and Synthesis
This compound features a pyridine ring substituted with a chlorine atom at the 4-position and a nitrile group at the 2-position. Its molecular formula is . The synthesis of this compound typically involves the cyanation of 4-chloropyridine N-oxide using trimethylsilanecarbonitrile (TMSCN) as a reagent, yielding high purity and yield suitable for further applications in drug development and material science.
Antimicrobial Properties
Research has indicated that derivatives of this compound exhibit varying degrees of antimicrobial activity. For instance, in a study evaluating several nitrogen heterocyclic compounds, derivatives including this compound showed weak tuberculostatic and antibacterial activities against strains of Mycobacterium tuberculosis and other bacteria. The minimum inhibitory concentrations (MICs) for these compounds ranged from 25 to 50 μg/cm³, which were notably less effective compared to standard treatments like isoniazid (INH) with MIC values of 0.5–1.0 μg/cm³ .
Cytotoxicity and Anticancer Potential
The cytotoxic effects of this compound derivatives have also been investigated. In vitro studies on various cancer cell lines demonstrated that some derivatives exhibited significant cytotoxicity. For example, one derivative showed an 88% growth inhibition at a concentration of 100 μg/cm³ against neonatal human dermal fibroblasts, while others displayed notable activity against melanoma (MALME-3M) and renal cancer (A498) cell lines . These findings suggest that modifications to the core structure can enhance anticancer properties.
Table: Summary of Biological Activities
The biological activity of this compound may be attributed to its ability to interact with various biological targets through its unique chemical structure, which allows for hydrogen bonding and π-stacking interactions. These interactions can facilitate the formation of stable complexes with biomolecules, potentially disrupting normal cellular functions or pathways involved in disease processes.
Q & A
Basic Research Questions
Q. What are the established synthetic routes for 4-Chloropyridine-2-carbonitrile, and how can purity be validated?
- Methodological Answer : The synthesis typically involves halogenation and cyanation of pyridine derivatives. For example, halogenation of pyridine precursors using chlorine donors (e.g., POCl₃) followed by nitrile introduction via nucleophilic substitution. Purity validation requires HPLC (High-Performance Liquid Chromatography) with UV detection, comparing retention times against standards. Cross-validate using melting point analysis and NMR spectroscopy (¹H/¹³C) to confirm structural integrity .
Q. Which spectroscopic and crystallographic techniques are critical for characterizing this compound?
- Methodological Answer : Use ¹H/¹³C NMR to confirm substituent positions and electronic environments. IR spectroscopy identifies the nitrile group (C≡N stretch ~2200 cm⁻¹). For crystallographic analysis, single-crystal X-ray diffraction (SCXRD) resolves molecular conformation and intermolecular interactions. Refinement software (e.g., SHELX) calculates bond angles and torsional strain, as demonstrated in studies of structurally related pyridine-carbonitriles .
Advanced Research Questions
Q. How can reaction yields be optimized for this compound under varying catalytic conditions?
- Methodological Answer : Systematic screening of catalysts (e.g., Pd/Cu for cross-coupling) and solvents (DMF, toluene) is essential. For example, Koutentis & Rees (2000) achieved higher yields using microwave-assisted synthesis to reduce side reactions. Design a fractional factorial experiment to test variables (temperature, catalyst loading) and analyze via ANOVA to identify significant factors .
Q. How should researchers resolve contradictions in reported biological activities of this compound derivatives?
- Methodological Answer : Discrepancies may arise from differences in assay conditions (e.g., cell lines, concentrations). Replicate experiments using standardized protocols (e.g., NIH/3T3 cells for cytotoxicity). Perform meta-analyses of published data, focusing on structure-activity relationships (SAR). For instance, El-Agrody et al. (2013) correlated anti-cancer activity with electron-withdrawing substituents at the pyridine ring .
Q. What safety protocols are recommended for handling this compound in laboratories?
- Methodological Answer : Use PPE (gloves, goggles, lab coats) and work in a fume hood. Avoid inhalation and skin contact due to potential toxicity (H313/H333 warnings). Waste must be segregated in labeled containers and processed by certified disposal services. Storage conditions should adhere to fire safety guidelines (away from ignition sources) .
Q. How can computational methods predict the reactivity of this compound in nucleophilic substitution reactions?
- Methodological Answer : Density Functional Theory (DFT) calculations (e.g., B3LYP/6-31G*) model electron density distributions to identify reactive sites. Molecular docking studies (AutoDock Vina) predict binding affinities with biological targets. Validate predictions experimentally via kinetic studies under controlled conditions .
Q. Data Analysis and Experimental Design
Q. What strategies mitigate variability in spectroscopic data for pyridine-carbonitrile derivatives?
- Methodological Answer : Calibrate instruments using certified reference materials. For NMR, employ deuterated solvents (e.g., DMSO-d₆) and internal standards (TMS). Use triplicate measurements and report standard deviations. Address solvent effects by comparing spectra in polar vs. non-polar solvents .
Q. How can crystallographic data inform the design of this compound-based inhibitors?
- Methodological Answer : SCXRD-derived bond lengths and angles reveal steric and electronic constraints. For example, the dihedral angle between chlorophenyl and pyridine rings impacts binding pocket compatibility. Overlay crystal structures with target proteins (e.g., kinases) using PyMOL to optimize inhibitor geometry .
Properties
IUPAC Name |
4-chloropyridine-2-carbonitrile | |
---|---|---|
Source | PubChem | |
URL | https://pubchem.ncbi.nlm.nih.gov | |
Description | Data deposited in or computed by PubChem | |
InChI |
InChI=1S/C6H3ClN2/c7-5-1-2-9-6(3-5)4-8/h1-3H | |
Source | PubChem | |
URL | https://pubchem.ncbi.nlm.nih.gov | |
Description | Data deposited in or computed by PubChem | |
InChI Key |
DYEZRXLVZMZHQT-UHFFFAOYSA-N | |
Source | PubChem | |
URL | https://pubchem.ncbi.nlm.nih.gov | |
Description | Data deposited in or computed by PubChem | |
Canonical SMILES |
C1=CN=C(C=C1Cl)C#N | |
Source | PubChem | |
URL | https://pubchem.ncbi.nlm.nih.gov | |
Description | Data deposited in or computed by PubChem | |
Molecular Formula |
C6H3ClN2 | |
Source | PubChem | |
URL | https://pubchem.ncbi.nlm.nih.gov | |
Description | Data deposited in or computed by PubChem | |
DSSTOX Substance ID |
DTXSID60351222 | |
Record name | 4-chloropyridine-2-carbonitrile | |
Source | EPA DSSTox | |
URL | https://comptox.epa.gov/dashboard/DTXSID60351222 | |
Description | DSSTox provides a high quality public chemistry resource for supporting improved predictive toxicology. | |
Molecular Weight |
138.55 g/mol | |
Source | PubChem | |
URL | https://pubchem.ncbi.nlm.nih.gov | |
Description | Data deposited in or computed by PubChem | |
CAS No. |
19235-89-3 | |
Record name | 4-Chloro-2-cyanopyridine | |
Source | CAS Common Chemistry | |
URL | https://commonchemistry.cas.org/detail?cas_rn=19235-89-3 | |
Description | CAS Common Chemistry is an open community resource for accessing chemical information. Nearly 500,000 chemical substances from CAS REGISTRY cover areas of community interest, including common and frequently regulated chemicals, and those relevant to high school and undergraduate chemistry classes. This chemical information, curated by our expert scientists, is provided in alignment with our mission as a division of the American Chemical Society. | |
Explanation | The data from CAS Common Chemistry is provided under a CC-BY-NC 4.0 license, unless otherwise stated. | |
Record name | 4-chloropyridine-2-carbonitrile | |
Source | EPA DSSTox | |
URL | https://comptox.epa.gov/dashboard/DTXSID60351222 | |
Description | DSSTox provides a high quality public chemistry resource for supporting improved predictive toxicology. | |
Synthesis routes and methods I
Procedure details
Synthesis routes and methods II
Procedure details
Synthesis routes and methods III
Procedure details
Synthesis routes and methods IV
Procedure details
Retrosynthesis Analysis
AI-Powered Synthesis Planning: Our tool employs the Template_relevance Pistachio, Template_relevance Bkms_metabolic, Template_relevance Pistachio_ringbreaker, Template_relevance Reaxys, Template_relevance Reaxys_biocatalysis model, leveraging a vast database of chemical reactions to predict feasible synthetic routes.
One-Step Synthesis Focus: Specifically designed for one-step synthesis, it provides concise and direct routes for your target compounds, streamlining the synthesis process.
Accurate Predictions: Utilizing the extensive PISTACHIO, BKMS_METABOLIC, PISTACHIO_RINGBREAKER, REAXYS, REAXYS_BIOCATALYSIS database, our tool offers high-accuracy predictions, reflecting the latest in chemical research and data.
Strategy Settings
Precursor scoring | Relevance Heuristic |
---|---|
Min. plausibility | 0.01 |
Model | Template_relevance |
Template Set | Pistachio/Bkms_metabolic/Pistachio_ringbreaker/Reaxys/Reaxys_biocatalysis |
Top-N result to add to graph | 6 |
Feasible Synthetic Routes
Disclaimer and Information on In-Vitro Research Products
Please be aware that all articles and product information presented on BenchChem are intended solely for informational purposes. The products available for purchase on BenchChem are specifically designed for in-vitro studies, which are conducted outside of living organisms. In-vitro studies, derived from the Latin term "in glass," involve experiments performed in controlled laboratory settings using cells or tissues. It is important to note that these products are not categorized as medicines or drugs, and they have not received approval from the FDA for the prevention, treatment, or cure of any medical condition, ailment, or disease. We must emphasize that any form of bodily introduction of these products into humans or animals is strictly prohibited by law. It is essential to adhere to these guidelines to ensure compliance with legal and ethical standards in research and experimentation.