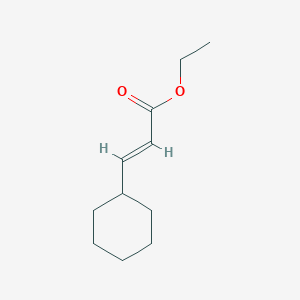
(E)-Ethyl 3-cyclohexylacrylate
Overview
Description
(E)-Ethyl 3-cyclohexylacrylate is an organic compound belonging to the family of acrylates. Acrylates are esters derived from acrylic acid and are known for their diverse applications in various fields. This compound is characterized by the presence of a cyclohexyl group attached to the acrylate moiety, which imparts unique chemical and physical properties.
Preparation Methods
Synthetic Routes and Reaction Conditions: (E)-Ethyl 3-cyclohexylacrylate can be synthesized through the esterification of acrylic acid with cyclohexanol in the presence of an acid catalyst. The reaction typically involves heating the reactants under reflux conditions to facilitate the formation of the ester bond. The reaction can be represented as follows:
Acrylic Acid+CyclohexanolAcid Catalyst(E)-Ethyl 3-cyclohexylacrylate+Water
Industrial Production Methods: In industrial settings, the production of this compound often involves the use of continuous flow reactors to ensure efficient mixing and heat transfer. The reaction conditions are optimized to achieve high yields and purity of the final product. The use of meso-structured silica materials as catalysts has been explored to enhance the selectivity and efficiency of the reaction .
Chemical Reactions Analysis
Types of Reactions: (E)-Ethyl 3-cyclohexylacrylate undergoes various chemical reactions, including:
Oxidation: The compound can be oxidized to form corresponding carboxylic acids or ketones.
Reduction: Reduction reactions can convert the ester group to alcohols.
Substitution: The acrylate moiety can undergo nucleophilic substitution reactions, leading to the formation of different derivatives.
Common Reagents and Conditions:
Oxidation: Common oxidizing agents include potassium permanganate and chromium trioxide.
Reduction: Reducing agents such as lithium aluminum hydride and sodium borohydride are used.
Substitution: Nucleophiles like amines and alcohols can react with the acrylate group under basic or acidic conditions.
Major Products Formed:
Oxidation: Carboxylic acids and ketones.
Reduction: Alcohols.
Substitution: Various substituted acrylates and derivatives.
Scientific Research Applications
(E)-Ethyl 3-cyclohexylacrylate has a wide range of applications in scientific research:
Mechanism of Action
The mechanism of action of (E)-Ethyl 3-cyclohexylacrylate involves its interaction with various molecular targets. The acrylate group is highly reactive and can undergo polymerization reactions, forming long chains of polymers. These polymers can interact with biological molecules, leading to changes in their structure and function. The cyclohexyl group provides hydrophobic interactions, enhancing the compound’s stability and compatibility with different substrates .
Comparison with Similar Compounds
- Methyl (E)-3-cyclohexylacrylate
- Butyl acrylate
- 2-Ethyl hexyl acrylate
Comparison: (E)-Ethyl 3-cyclohexylacrylate is unique due to the presence of the cyclohexyl group, which imparts specific hydrophobic properties and enhances its stability. Compared to other acrylates like butyl acrylate and 2-ethyl hexyl acrylate, this compound offers better film-forming properties and is more suitable for applications requiring high durability and resistance .
Properties
IUPAC Name |
ethyl (E)-3-cyclohexylprop-2-enoate | |
---|---|---|
Source | PubChem | |
URL | https://pubchem.ncbi.nlm.nih.gov | |
Description | Data deposited in or computed by PubChem | |
InChI |
InChI=1S/C11H18O2/c1-2-13-11(12)9-8-10-6-4-3-5-7-10/h8-10H,2-7H2,1H3/b9-8+ | |
Source | PubChem | |
URL | https://pubchem.ncbi.nlm.nih.gov | |
Description | Data deposited in or computed by PubChem | |
InChI Key |
SLGCKLRNFQEFEO-CMDGGOBGSA-N | |
Source | PubChem | |
URL | https://pubchem.ncbi.nlm.nih.gov | |
Description | Data deposited in or computed by PubChem | |
Canonical SMILES |
CCOC(=O)C=CC1CCCCC1 | |
Source | PubChem | |
URL | https://pubchem.ncbi.nlm.nih.gov | |
Description | Data deposited in or computed by PubChem | |
Isomeric SMILES |
CCOC(=O)/C=C/C1CCCCC1 | |
Source | PubChem | |
URL | https://pubchem.ncbi.nlm.nih.gov | |
Description | Data deposited in or computed by PubChem | |
Molecular Formula |
C11H18O2 | |
Source | PubChem | |
URL | https://pubchem.ncbi.nlm.nih.gov | |
Description | Data deposited in or computed by PubChem | |
Molecular Weight |
182.26 g/mol | |
Source | PubChem | |
URL | https://pubchem.ncbi.nlm.nih.gov | |
Description | Data deposited in or computed by PubChem | |
CAS No. |
17343-88-3 | |
Record name | NSC244952 | |
Source | DTP/NCI | |
URL | https://dtp.cancer.gov/dtpstandard/servlet/dwindex?searchtype=NSC&outputformat=html&searchlist=244952 | |
Description | The NCI Development Therapeutics Program (DTP) provides services and resources to the academic and private-sector research communities worldwide to facilitate the discovery and development of new cancer therapeutic agents. | |
Explanation | Unless otherwise indicated, all text within NCI products is free of copyright and may be reused without our permission. Credit the National Cancer Institute as the source. | |
Synthesis routes and methods I
Procedure details
Synthesis routes and methods II
Procedure details
Retrosynthesis Analysis
AI-Powered Synthesis Planning: Our tool employs the Template_relevance Pistachio, Template_relevance Bkms_metabolic, Template_relevance Pistachio_ringbreaker, Template_relevance Reaxys, Template_relevance Reaxys_biocatalysis model, leveraging a vast database of chemical reactions to predict feasible synthetic routes.
One-Step Synthesis Focus: Specifically designed for one-step synthesis, it provides concise and direct routes for your target compounds, streamlining the synthesis process.
Accurate Predictions: Utilizing the extensive PISTACHIO, BKMS_METABOLIC, PISTACHIO_RINGBREAKER, REAXYS, REAXYS_BIOCATALYSIS database, our tool offers high-accuracy predictions, reflecting the latest in chemical research and data.
Strategy Settings
Precursor scoring | Relevance Heuristic |
---|---|
Min. plausibility | 0.01 |
Model | Template_relevance |
Template Set | Pistachio/Bkms_metabolic/Pistachio_ringbreaker/Reaxys/Reaxys_biocatalysis |
Top-N result to add to graph | 6 |
Feasible Synthetic Routes
Disclaimer and Information on In-Vitro Research Products
Please be aware that all articles and product information presented on BenchChem are intended solely for informational purposes. The products available for purchase on BenchChem are specifically designed for in-vitro studies, which are conducted outside of living organisms. In-vitro studies, derived from the Latin term "in glass," involve experiments performed in controlled laboratory settings using cells or tissues. It is important to note that these products are not categorized as medicines or drugs, and they have not received approval from the FDA for the prevention, treatment, or cure of any medical condition, ailment, or disease. We must emphasize that any form of bodily introduction of these products into humans or animals is strictly prohibited by law. It is essential to adhere to these guidelines to ensure compliance with legal and ethical standards in research and experimentation.