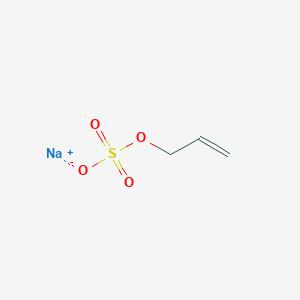
Allyl sodium sulfate
Overview
Description
Allyl sodium sulfate, also known as sodium allylsulfonate, is a unique chemical compound . It is provided to early discovery researchers as part of a collection of unique chemicals . Its empirical formula is C3H5NaO3S and it has a molecular weight of 144.12 .
Synthesis Analysis
The synthesis of sodium sulfinates, which includes this compound, has been extensively studied . Sodium sulfinates act as versatile building blocks for preparing many valuable organosulfur compounds through S–S, N–S, and C–S bond-forming reactions . A synthetic method of Sodium Allyl Sulfonate includes adding phase transfer catalyst and polymerization inhibitor into sodium metabisulfite solution, uniformly mixing to obtain sodium pyrosulfite mixed liquor .
Molecular Structure Analysis
The molecular formula of this compound is C3H5NaO4S . Its average mass is 160.124 Da and its monoisotopic mass is 159.980621 Da .
Chemical Reactions Analysis
Allyl halides and tosylates, which are related to this compound, are excellent electrophiles for bimolecular nucleophilic substitution reactions (SN2) . They exhibit faster SN2 reactivity than secondary alkyl halides because the bimolecular transition state is stabilized by hyperconjugation between the orbital of the nucleophile and the conjugated pi bond of the allylic group .
Scientific Research Applications
Micellar Properties and Photophysical Methods Research by Romani, Gehlen, and Quina (2001) explored the properties of sodium dodecyl sulfate micelles in the presence of isomeric and unsaturated short-chain alcohols, including allyl alcohol. This study contributes to understanding the behavior of micelles, which are critical in many industrial and research applications, such as drug delivery systems and as models for biological membranes (Romani, Gehlen, & Quina, 2001).
Technological Improvements in Chemical Processes Zhang Chen-dong (2008) discussed the use of sodium allylsulfonate in improving the yield of lead salt in the production of sodium 2,3-dimercaptopropane-1-sulfonate. This study highlights the role of allyl sodium sulfate in enhancing chemical synthesis processes, particularly in the context of heavy metal salt poisoning treatment (Zhang Chen-dong, 2008).
Carbon Nanotube Separation A study by Liu, Tanaka, Urabe, and Kataura (2013) demonstrated the application of temperature-controlled gel chromatography using allyl dextran-based gel for the single-chirality separation of single-wall carbon nanotubes. This innovative method has significant implications for the industrial-scale separation of carbon nanotubes, a critical aspect of nanotechnology and materials science (Liu, Tanaka, Urabe, & Kataura, 2013).
Environmental Applications in Water Treatment The research by Saitoh, Fukushima, and Miwa (2014) investigated the combined use of surfactant-induced coagulation of poly(allylamine hydrochloride) for rapid removal of estrogens and phenolic compounds from water. This study provides valuable insights into the potential environmental applications of allyl-based compounds in water purification and waste treatment processes (Saitoh, Fukushima, & Miwa, 2014).
Mechanism of Action
While the exact mechanism of action of Allyl sodium sulfate is not well-studied, related compounds like Sodium sulfate have been studied . Sodium sulfate anhydrous disassociates in water to provide sodium ions and sulfate ions. Sodium ion is the principal cation of the extracellular fluid and plays a large part in the therapy of fluid and electrolyte disturbances .
Safety and Hazards
Future Directions
While specific future directions for Allyl sodium sulfate are not well-documented, related compounds like Allyl Isothiocyanate have been studied for their potential therapeutic effects . For instance, Allyl Isothiocyanate has been found to ameliorate Dextran Sodium Sulfate-Induced Colitis in mice by enhancing tight junction and mucin expression . This suggests potential future directions for the study of this compound and related compounds in therapeutic contexts.
properties
IUPAC Name |
sodium;prop-2-enyl sulfate | |
---|---|---|
Source | PubChem | |
URL | https://pubchem.ncbi.nlm.nih.gov | |
Description | Data deposited in or computed by PubChem | |
InChI |
InChI=1S/C3H6O4S.Na/c1-2-3-7-8(4,5)6;/h2H,1,3H2,(H,4,5,6);/q;+1/p-1 | |
Source | PubChem | |
URL | https://pubchem.ncbi.nlm.nih.gov | |
Description | Data deposited in or computed by PubChem | |
InChI Key |
USEWKBLLMCFAEJ-UHFFFAOYSA-M | |
Source | PubChem | |
URL | https://pubchem.ncbi.nlm.nih.gov | |
Description | Data deposited in or computed by PubChem | |
Canonical SMILES |
C=CCOS(=O)(=O)[O-].[Na+] | |
Source | PubChem | |
URL | https://pubchem.ncbi.nlm.nih.gov | |
Description | Data deposited in or computed by PubChem | |
Molecular Formula |
C3H5NaO4S | |
Source | PubChem | |
URL | https://pubchem.ncbi.nlm.nih.gov | |
Description | Data deposited in or computed by PubChem | |
DSSTOX Substance ID |
DTXSID80172517 | |
Record name | Sulfuric acid, mono-2-propenyl ester, sodium salt | |
Source | EPA DSSTox | |
URL | https://comptox.epa.gov/dashboard/DTXSID80172517 | |
Description | DSSTox provides a high quality public chemistry resource for supporting improved predictive toxicology. | |
Molecular Weight |
160.13 g/mol | |
Source | PubChem | |
URL | https://pubchem.ncbi.nlm.nih.gov | |
Description | Data deposited in or computed by PubChem | |
CAS RN |
19037-59-3 | |
Record name | Sulfuric acid, mono-2-propenyl ester, sodium salt | |
Source | ChemIDplus | |
URL | https://pubchem.ncbi.nlm.nih.gov/substance/?source=chemidplus&sourceid=0019037593 | |
Description | ChemIDplus is a free, web search system that provides access to the structure and nomenclature authority files used for the identification of chemical substances cited in National Library of Medicine (NLM) databases, including the TOXNET system. | |
Record name | Sulfuric acid, mono-2-propenyl ester, sodium salt | |
Source | EPA DSSTox | |
URL | https://comptox.epa.gov/dashboard/DTXSID80172517 | |
Description | DSSTox provides a high quality public chemistry resource for supporting improved predictive toxicology. | |
Retrosynthesis Analysis
AI-Powered Synthesis Planning: Our tool employs the Template_relevance Pistachio, Template_relevance Bkms_metabolic, Template_relevance Pistachio_ringbreaker, Template_relevance Reaxys, Template_relevance Reaxys_biocatalysis model, leveraging a vast database of chemical reactions to predict feasible synthetic routes.
One-Step Synthesis Focus: Specifically designed for one-step synthesis, it provides concise and direct routes for your target compounds, streamlining the synthesis process.
Accurate Predictions: Utilizing the extensive PISTACHIO, BKMS_METABOLIC, PISTACHIO_RINGBREAKER, REAXYS, REAXYS_BIOCATALYSIS database, our tool offers high-accuracy predictions, reflecting the latest in chemical research and data.
Strategy Settings
Precursor scoring | Relevance Heuristic |
---|---|
Min. plausibility | 0.01 |
Model | Template_relevance |
Template Set | Pistachio/Bkms_metabolic/Pistachio_ringbreaker/Reaxys/Reaxys_biocatalysis |
Top-N result to add to graph | 6 |
Feasible Synthetic Routes
Disclaimer and Information on In-Vitro Research Products
Please be aware that all articles and product information presented on BenchChem are intended solely for informational purposes. The products available for purchase on BenchChem are specifically designed for in-vitro studies, which are conducted outside of living organisms. In-vitro studies, derived from the Latin term "in glass," involve experiments performed in controlled laboratory settings using cells or tissues. It is important to note that these products are not categorized as medicines or drugs, and they have not received approval from the FDA for the prevention, treatment, or cure of any medical condition, ailment, or disease. We must emphasize that any form of bodily introduction of these products into humans or animals is strictly prohibited by law. It is essential to adhere to these guidelines to ensure compliance with legal and ethical standards in research and experimentation.