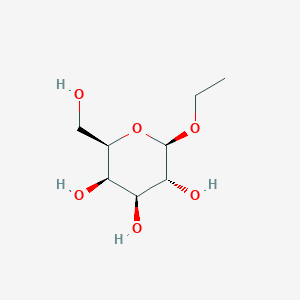
Ethyl Beta-D-Galactopyranoside
Overview
Description
Scientific Research Applications
Eleutheroside C has a wide range of applications in scientific research:
Chemistry: Used as a reference compound in chromatography for the identification of Eleutherococcus senticosus extracts.
Biology: Studied for its potential effects on cellular processes and its role in plant metabolism.
Medicine: Investigated for its adaptogenic properties, which may help the body resist stressors of various kinds.
Mechanism of Action
The mechanism by which Eleutheroside C exerts its effects is not fully understood, but it is believed to interact with various molecular targets and pathways:
Molecular Targets: Potential targets include enzymes involved in stress response and metabolic pathways.
Pathways Involved: It may modulate pathways related to oxidative stress, inflammation, and energy metabolism, contributing to its adaptogenic effects.
Biochemical Analysis
Biochemical Properties
Ethyl Beta-D-Galactopyranoside interacts with a variety of enzymes, proteins, and other biomolecules. For instance, it is a substrate for the enzyme β-D-Galactosidase, which catalyzes the hydrolysis of the glycosidic oxygen link between the terminal non-reducing D-galactoside unit and the glycoside molecule . This reaction is crucial in various biochemical pathways and has applications in food pasteurization, biomass conversion, and more .
Cellular Effects
The effects of this compound on cells and cellular processes are diverse. It influences cell function by interacting with various cell signaling pathways, gene expression, and cellular metabolism . For instance, it has been found to have antifungal activities, indicating its potential role in cellular defense mechanisms .
Molecular Mechanism
At the molecular level, this compound exerts its effects through binding interactions with biomolecules, enzyme inhibition or activation, and changes in gene expression . For example, it has been shown to have higher binding energy than the antifungal drug, fluconazole, when interacting with the enzyme sterol 14α-demethylase .
Temporal Effects in Laboratory Settings
In laboratory settings, the effects of this compound change over time. It has been observed that the compound is thermally stable up to 270 °C . Moreover, its effects on cellular function, such as its antifungal activity, can be observed in both in vitro and in vivo studies over extended periods .
Metabolic Pathways
This compound is involved in several metabolic pathways. It interacts with enzymes such as β-D-Galactosidase and influences metabolic flux and metabolite levels
Preparation Methods
Synthetic Routes and Reaction Conditions: Eleutheroside C can be synthesized through the glycosylation of galactose with ethanol under acidic conditions. The reaction typically involves the use of a catalyst such as sulfuric acid or hydrochloric acid to facilitate the formation of the glycosidic bond.
Industrial Production Methods: In an industrial setting, Eleutheroside C is usually extracted from the roots of Eleutherococcus senticosus. The extraction process involves several steps:
Harvesting and Drying: The roots are harvested and dried to reduce moisture content.
Grinding: The dried roots are ground into a fine powder.
Extraction: The powder is subjected to solvent extraction using ethanol or methanol.
Purification: The extract is purified using techniques such as column chromatography to isolate Eleutheroside C.
Types of Reactions:
Oxidation: Eleutheroside C can undergo oxidation reactions, typically in the presence of strong oxidizing agents like potassium permanganate or hydrogen peroxide.
Reduction: Reduction reactions can be carried out using reducing agents such as sodium borohydride.
Substitution: Substitution reactions may involve the replacement of the ethyl group with other alkyl groups under specific conditions.
Common Reagents and Conditions:
Oxidation: Potassium permanganate, hydrogen peroxide.
Reduction: Sodium borohydride, lithium aluminum hydride.
Substitution: Alkyl halides, in the presence of a base like sodium hydroxide.
Major Products:
Oxidation: Oxidized derivatives of Eleutheroside C.
Reduction: Reduced forms of Eleutheroside C.
Substitution: Various alkylated derivatives depending on the substituent used.
Comparison with Similar Compounds
Eleutheroside C is unique among eleutherosides due to its specific glycosidic structure. Similar compounds include:
Eleutheroside A (daucosterol): A saponin and sterol glycoside.
Eleutheroside B (syringin): A phenylpropanoid glycoside.
Eleutheroside E: An optical isomer of acanthoside D.
Compared to these compounds, Eleutheroside C is distinguished by its ethyl galactoside structure, which may confer different biological activities and pharmacokinetic properties .
properties
IUPAC Name |
(2S,3R,4S,5R,6R)-2-ethoxy-6-(hydroxymethyl)oxane-3,4,5-triol | |
---|---|---|
Source | PubChem | |
URL | https://pubchem.ncbi.nlm.nih.gov | |
Description | Data deposited in or computed by PubChem | |
InChI |
InChI=1S/C8H16O6/c1-2-13-8-7(12)6(11)5(10)4(3-9)14-8/h4-12H,2-3H2,1H3/t4-,5+,6+,7-,8+/m1/s1 | |
Source | PubChem | |
URL | https://pubchem.ncbi.nlm.nih.gov | |
Description | Data deposited in or computed by PubChem | |
InChI Key |
WYUFTYLVLQZQNH-HNEXDWKRSA-N | |
Source | PubChem | |
URL | https://pubchem.ncbi.nlm.nih.gov | |
Description | Data deposited in or computed by PubChem | |
Canonical SMILES |
CCOC1C(C(C(C(O1)CO)O)O)O | |
Source | PubChem | |
URL | https://pubchem.ncbi.nlm.nih.gov | |
Description | Data deposited in or computed by PubChem | |
Isomeric SMILES |
CCO[C@@H]1[C@@H]([C@H]([C@H]([C@H](O1)CO)O)O)O | |
Source | PubChem | |
URL | https://pubchem.ncbi.nlm.nih.gov | |
Description | Data deposited in or computed by PubChem | |
Molecular Formula |
C8H16O6 | |
Record name | Eleutheroside C | |
Source | Wikipedia | |
URL | https://en.wikipedia.org/wiki/Dictionary_of_chemical_formulas | |
Description | Chemical information link to Wikipedia. | |
Source | PubChem | |
URL | https://pubchem.ncbi.nlm.nih.gov | |
Description | Data deposited in or computed by PubChem | |
DSSTOX Substance ID |
DTXSID50879263 | |
Record name | ?-D-GALACTOPYRANOSIDE, ETHYL | |
Source | EPA DSSTox | |
URL | https://comptox.epa.gov/dashboard/DTXSID50879263 | |
Description | DSSTox provides a high quality public chemistry resource for supporting improved predictive toxicology. | |
Molecular Weight |
208.21 g/mol | |
Source | PubChem | |
URL | https://pubchem.ncbi.nlm.nih.gov | |
Description | Data deposited in or computed by PubChem | |
CAS RN |
18997-88-1 | |
Record name | ?-D-GALACTOPYRANOSIDE, ETHYL | |
Source | EPA DSSTox | |
URL | https://comptox.epa.gov/dashboard/DTXSID50879263 | |
Description | DSSTox provides a high quality public chemistry resource for supporting improved predictive toxicology. | |
Retrosynthesis Analysis
AI-Powered Synthesis Planning: Our tool employs the Template_relevance Pistachio, Template_relevance Bkms_metabolic, Template_relevance Pistachio_ringbreaker, Template_relevance Reaxys, Template_relevance Reaxys_biocatalysis model, leveraging a vast database of chemical reactions to predict feasible synthetic routes.
One-Step Synthesis Focus: Specifically designed for one-step synthesis, it provides concise and direct routes for your target compounds, streamlining the synthesis process.
Accurate Predictions: Utilizing the extensive PISTACHIO, BKMS_METABOLIC, PISTACHIO_RINGBREAKER, REAXYS, REAXYS_BIOCATALYSIS database, our tool offers high-accuracy predictions, reflecting the latest in chemical research and data.
Strategy Settings
Precursor scoring | Relevance Heuristic |
---|---|
Min. plausibility | 0.01 |
Model | Template_relevance |
Template Set | Pistachio/Bkms_metabolic/Pistachio_ringbreaker/Reaxys/Reaxys_biocatalysis |
Top-N result to add to graph | 6 |
Feasible Synthetic Routes
Q & A
Q1: What is the significance of studying the reaction of Ethyl Beta-D-Galactopyranoside with beta-galactosidase?
A1: Studying the reaction of this compound with beta-galactosidase helps us understand the enzyme's mechanism of action and its substrate specificity. [] Beta-galactosidase is a crucial enzyme involved in the breakdown of lactose, and understanding its interaction with different substrates like this compound provides valuable insights into carbohydrate metabolism and enzyme kinetics. []
Q2: How does the structure of this compound influence its reactivity with beta-galactosidase?
A2: Research suggests that the basicity of the alkoxy group in alkyl Beta-D-Galactopyranosides, like this compound, plays a key role in their reactivity with beta-galactosidase. [] As the basicity of the alkoxy group decreases, the alkyl Beta-D-Galactopyranoside becomes easier to cleave by the enzyme. [] This information is crucial for understanding the structure-activity relationship of beta-galactosidase substrates and designing potential inhibitors or activators.
Q3: Can you explain the significance of the Brønsted parameter in the context of this compound and beta-galactosidase?
A3: The Brønsted parameter (β) helps quantify the sensitivity of a reaction rate to changes in the pKa of reactants. In the case of this compound and beta-galactosidase, a βnuc value of -0.19 ± 0.10 was determined for the reaction of alcohols with the galactosylated enzyme intermediate. [] This negative value indicates that the reaction rate increases with decreasing pKa of the alcohol, suggesting that the alcohol acts as a nucleophile in the reaction. []
Q4: How does the use of this compound in liposome studies contribute to drug delivery research?
A4: this compound can be incorporated into liposomes, which are artificial vesicles used for drug delivery. [, ] The galactose moiety on this compound can be recognized by specific receptors, such as the asialoglycoprotein receptor found on hepatocytes (liver cells). [] This targeted recognition can potentially be exploited for the delivery of drugs specifically to the liver. []
Q5: The research mentions using a lipophilic radical initiator to create galactose-containing amphiphiles. What is the advantage of this approach?
A5: Using a lipophilic radical initiator allows for the polymerization of galactose-containing monomers like 2-(methacryloyloxy)this compound. [] This polymerization creates amphiphiles with varying degrees of polymerization, which influences their ability to form liposomes and interact with biological systems. [, ] Controlling the degree of polymerization allows researchers to fine-tune the properties of the resulting liposomes for better stability and targeted drug delivery applications. [, ]
Disclaimer and Information on In-Vitro Research Products
Please be aware that all articles and product information presented on BenchChem are intended solely for informational purposes. The products available for purchase on BenchChem are specifically designed for in-vitro studies, which are conducted outside of living organisms. In-vitro studies, derived from the Latin term "in glass," involve experiments performed in controlled laboratory settings using cells or tissues. It is important to note that these products are not categorized as medicines or drugs, and they have not received approval from the FDA for the prevention, treatment, or cure of any medical condition, ailment, or disease. We must emphasize that any form of bodily introduction of these products into humans or animals is strictly prohibited by law. It is essential to adhere to these guidelines to ensure compliance with legal and ethical standards in research and experimentation.