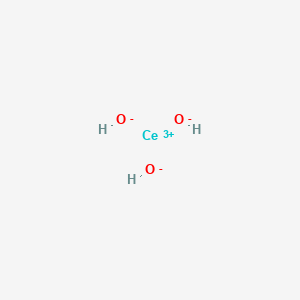
Cerium hydroxide
Overview
Description
Cerium hydroxide is an inorganic compound with the chemical formula Ce(OH)₃. It is a rare earth hydroxide that appears as a white or pale yellow powder. This compound is primarily used as a precursor to other cerium compounds and has various applications in catalysis, glass manufacturing, and as a polishing agent.
Preparation Methods
Synthetic Routes and Reaction Conditions: Cerium hydroxide can be synthesized through several methods, including:
Precipitation Method: This involves the reaction of cerium salts, such as cerium nitrate or cerium chloride, with a strong base like sodium hydroxide or potassium hydroxide. The reaction typically occurs at room temperature and results in the formation of this compound precipitate.
Hydrothermal Synthesis: This method involves the reaction of cerium salts with a base under high temperature and pressure conditions. The hydrothermal method can produce this compound with controlled particle size and morphology.
Industrial Production Methods:
Radiation-Induced Formation: this compound nanoparticles can be formed by radiation-mediated increase in local pH.
Green Synthesis: this compound can also be prepared using environmentally friendly methods, such as the reaction of cerium carbonate with water under mild conditions.
Types of Reactions:
Oxidation: this compound can undergo oxidation to form cerium oxide (CeO₂). This reaction is typically carried out by heating this compound in the presence of oxygen.
Reduction: this compound can be reduced to cerium metal under specific conditions, such as in the presence of a strong reducing agent.
Substitution: this compound can react with various acids to form cerium salts. For example, reacting this compound with hydrochloric acid produces cerium chloride.
Common Reagents and Conditions:
Oxidation: Oxygen or air at elevated temperatures.
Reduction: Strong reducing agents like hydrogen gas.
Substitution: Acids such as hydrochloric acid, sulfuric acid, or nitric acid.
Major Products Formed:
Oxidation: Cerium oxide (CeO₂).
Reduction: Cerium metal.
Substitution: Cerium salts like cerium chloride, cerium sulfate, and cerium nitrate.
Scientific Research Applications
Cerium hydroxide has a wide range of applications in scientific research, including:
Catalysis: this compound is used as a catalyst or catalyst support in various chemical reactions, including oxidation and reduction reactions.
Glass Manufacturing: It is used in the production of specialized glass, such as glass for optical lenses and precision optics, due to its polishing properties.
Biomedical Applications: this compound nanoparticles have shown potential in biomedical applications, including as antioxidant agents and in drug delivery systems.
Environmental Applications: this compound is used in environmental remediation processes, such as the removal of pollutants from water and air.
Mechanism of Action
The mechanism of action of cerium hydroxide involves its ability to undergo redox reactions. This compound can switch between the Ce³⁺ and Ce⁴⁺ oxidation states, allowing it to participate in various redox processes. This redox activity is crucial for its catalytic properties and its ability to scavenge free radicals in biomedical applications .
Comparison with Similar Compounds
Lanthanum Hydroxide (La(OH)₃): Similar in structure but less effective in redox reactions.
Praseodymium Hydroxide (Pr(OH)₃): Similar in structure but has different redox properties compared to cerium hydroxide.
This compound’s unique redox properties and wide range of applications make it a valuable compound in various scientific and industrial fields.
Biological Activity
Cerium hydroxide (Ce(OH)₃) is a compound of cerium that has garnered attention for its biological activity, particularly in biomedical applications. This article explores the various aspects of this compound's biological activity, including its antimicrobial properties, antioxidant effects, and potential therapeutic applications. The information is supported by case studies and research findings from diverse sources.
Overview of this compound
This compound exists primarily in two forms: cerium(III) hydroxide and cerium(IV) hydroxide. The former is more soluble and is often studied for its biological properties. Cerium compounds are known for their ability to switch between oxidation states (Ce³⁺ and Ce⁴⁺), which plays a crucial role in their reactivity and biological interactions.
Antimicrobial Properties
This compound nanoparticles (CeNPs) exhibit significant antimicrobial activity against various pathogens. Studies have shown that these nanoparticles can inhibit the growth of both Gram-positive and Gram-negative bacteria.
Key Findings:
- Inhibition Mechanism: CeNPs interact with bacterial cells through adsorption, leading to oxidative stress due to the generation of reactive oxygen species (ROS). This interaction does not penetrate the bacterial cell but modifies surface properties, affecting cellular transport and metabolism .
- Efficacy: Research indicates that cerium oxide nanoparticles can achieve up to 100% inhibition of microbial cell viability at concentrations as low as 500 mg/L. They have shown effectiveness against pathogens such as Staphylococcus aureus and Pseudomonas aeruginosa .
Antioxidant Activity
This compound demonstrates notable antioxidant properties, functioning as a superoxide dismutase (SOD) mimetic. This property allows it to scavenge free radicals and mitigate oxidative stress in biological systems.
Mechanism of Action:
- The autoregenerative cycle of cerium compounds enables them to absorb or donate electrons from active oxygen species, thus neutralizing their harmful effects .
- In vitro studies have shown that CeNPs can enhance cell viability under oxidative stress conditions induced by hydrogen peroxide (H₂O₂), indicating their potential for therapeutic applications in oxidative stress-related diseases .
Case Studies
-
Cell Viability and Differentiation:
A study evaluated the biocompatibility of CeO₂ nanoparticles with human periodontal ligament cells (hPDLCs). Results indicated that the presence of CeO₂ enhanced cell proliferation and osteogenic differentiation markers, suggesting potential applications in dental tissue engineering . -
Toxicity Assessment:
A stereological study assessed the toxic effects of cerium oxide on fetal renal development in pregnant mice. High doses were found to impair kidney development, highlighting the need for careful dosage consideration in therapeutic applications .
Research Findings Summary Table
Properties
IUPAC Name |
cerium(3+);trihydroxide | |
---|---|---|
Source | PubChem | |
URL | https://pubchem.ncbi.nlm.nih.gov | |
Description | Data deposited in or computed by PubChem | |
InChI |
InChI=1S/Ce.3H2O/h;3*1H2/q+3;;;/p-3 | |
Source | PubChem | |
URL | https://pubchem.ncbi.nlm.nih.gov | |
Description | Data deposited in or computed by PubChem | |
InChI Key |
UNJPQTDTZAKTFK-UHFFFAOYSA-K | |
Source | PubChem | |
URL | https://pubchem.ncbi.nlm.nih.gov | |
Description | Data deposited in or computed by PubChem | |
Canonical SMILES |
[OH-].[OH-].[OH-].[Ce+3] | |
Source | PubChem | |
URL | https://pubchem.ncbi.nlm.nih.gov | |
Description | Data deposited in or computed by PubChem | |
Molecular Formula |
Ce(OH)3, CeH3O3 | |
Record name | Cerium(III) hydroxide | |
Source | Wikipedia | |
URL | https://en.wikipedia.org/wiki/Cerium(III)_hydroxide | |
Description | Chemical information link to Wikipedia. | |
Source | PubChem | |
URL | https://pubchem.ncbi.nlm.nih.gov | |
Description | Data deposited in or computed by PubChem | |
DSSTOX Substance ID |
DTXSID9065942 | |
Record name | Cerium(III) hydroxide | |
Source | EPA DSSTox | |
URL | https://comptox.epa.gov/dashboard/DTXSID9065942 | |
Description | DSSTox provides a high quality public chemistry resource for supporting improved predictive toxicology. | |
Molecular Weight |
191.138 g/mol | |
Source | PubChem | |
URL | https://pubchem.ncbi.nlm.nih.gov | |
Description | Data deposited in or computed by PubChem | |
Physical Description |
White solid; Yellow, brown, or pink if impure; [Hawley] | |
Record name | Cerous hydroxide | |
Source | Haz-Map, Information on Hazardous Chemicals and Occupational Diseases | |
URL | https://haz-map.com/Agents/9110 | |
Description | Haz-Map® is an occupational health database designed for health and safety professionals and for consumers seeking information about the adverse effects of workplace exposures to chemical and biological agents. | |
Explanation | Copyright (c) 2022 Haz-Map(R). All rights reserved. Unless otherwise indicated, all materials from Haz-Map are copyrighted by Haz-Map(R). No part of these materials, either text or image may be used for any purpose other than for personal use. Therefore, reproduction, modification, storage in a retrieval system or retransmission, in any form or by any means, electronic, mechanical or otherwise, for reasons other than personal use, is strictly prohibited without prior written permission. | |
CAS No. |
15785-09-8 | |
Record name | Cerium hydroxide (Ce(OH)3) | |
Source | CAS Common Chemistry | |
URL | https://commonchemistry.cas.org/detail?cas_rn=15785-09-8 | |
Description | CAS Common Chemistry is an open community resource for accessing chemical information. Nearly 500,000 chemical substances from CAS REGISTRY cover areas of community interest, including common and frequently regulated chemicals, and those relevant to high school and undergraduate chemistry classes. This chemical information, curated by our expert scientists, is provided in alignment with our mission as a division of the American Chemical Society. | |
Explanation | The data from CAS Common Chemistry is provided under a CC-BY-NC 4.0 license, unless otherwise stated. | |
Record name | Cerium hydroxide (Ce(OH)3) | |
Source | ChemIDplus | |
URL | https://pubchem.ncbi.nlm.nih.gov/substance/?source=chemidplus&sourceid=0015785098 | |
Description | ChemIDplus is a free, web search system that provides access to the structure and nomenclature authority files used for the identification of chemical substances cited in National Library of Medicine (NLM) databases, including the TOXNET system. | |
Record name | Cerium hydroxide (Ce(OH)3) | |
Source | EPA Chemicals under the TSCA | |
URL | https://www.epa.gov/chemicals-under-tsca | |
Description | EPA Chemicals under the Toxic Substances Control Act (TSCA) collection contains information on chemicals and their regulations under TSCA, including non-confidential content from the TSCA Chemical Substance Inventory and Chemical Data Reporting. | |
Record name | Cerium(III) hydroxide | |
Source | EPA DSSTox | |
URL | https://comptox.epa.gov/dashboard/DTXSID9065942 | |
Description | DSSTox provides a high quality public chemistry resource for supporting improved predictive toxicology. | |
Record name | Cerium trihydroxide | |
Source | European Chemicals Agency (ECHA) | |
URL | https://echa.europa.eu/substance-information/-/substanceinfo/100.036.241 | |
Description | The European Chemicals Agency (ECHA) is an agency of the European Union which is the driving force among regulatory authorities in implementing the EU's groundbreaking chemicals legislation for the benefit of human health and the environment as well as for innovation and competitiveness. | |
Explanation | Use of the information, documents and data from the ECHA website is subject to the terms and conditions of this Legal Notice, and subject to other binding limitations provided for under applicable law, the information, documents and data made available on the ECHA website may be reproduced, distributed and/or used, totally or in part, for non-commercial purposes provided that ECHA is acknowledged as the source: "Source: European Chemicals Agency, http://echa.europa.eu/". Such acknowledgement must be included in each copy of the material. ECHA permits and encourages organisations and individuals to create links to the ECHA website under the following cumulative conditions: Links can only be made to webpages that provide a link to the Legal Notice page. | |
Disclaimer and Information on In-Vitro Research Products
Please be aware that all articles and product information presented on BenchChem are intended solely for informational purposes. The products available for purchase on BenchChem are specifically designed for in-vitro studies, which are conducted outside of living organisms. In-vitro studies, derived from the Latin term "in glass," involve experiments performed in controlled laboratory settings using cells or tissues. It is important to note that these products are not categorized as medicines or drugs, and they have not received approval from the FDA for the prevention, treatment, or cure of any medical condition, ailment, or disease. We must emphasize that any form of bodily introduction of these products into humans or animals is strictly prohibited by law. It is essential to adhere to these guidelines to ensure compliance with legal and ethical standards in research and experimentation.