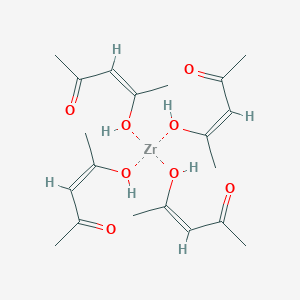
Zirconium acetylacetonate
Overview
Description
Zirconium acetylacetonate is a coordination complex with the formula Zr(C5H7O2)4 . It is a common acetylacetonate of zirconium and is a white solid .
Synthesis Analysis
The complex is prepared by treating zirconium oxychloride with acetylacetone . The synthesis can involve the reaction of zinc acetylacetonate hydrate, aluminium acetylacetonate, and 1,2-hexadecanediol in the presence of oleic acid and oleyl amine .Molecular Structure Analysis
The molecular weight of Zirconium acetylacetonate is 487.656 . The complex has a square antiprismatic geometry with eight nearly equivalent Zr-O bonds of length 2.19 Å .Chemical Reactions Analysis
Zirconium(IV) acetylacetonate is a very useful initiator of lactide polymerization and copolymerization, lactides with lactone copolymerization reactions . The thermal decomposition of Zr(acac)4 is studied in a SiC-microreactor on the micro-second time scale .Physical And Chemical Properties Analysis
Zirconium acetylacetonate is a white solid that exhibits high solubility in nonpolar organic solvents, but not simple hydrocarbons . The molecular weight of Zirconium acetylacetonate is 487.656 .Scientific Research Applications
Synthesis of Zirconia Nanoparticles
Zirconium acetylacetonate can be used as a sol-gel precursor to synthesize zirconia nanoparticles . These nanoparticles have photocatalytic activity, which can be utilized in various fields such as environmental remediation and solar energy conversion .
Improvement of Perovskite Solar Cells
This compound can be used as an additive to improve the performance of the electron transport layer in perovskite solar cells . This can enhance the efficiency and stability of these solar cells .
Catalyst in Organic Syntheses
Zirconium acetylacetonate is commonly used in various catalysts and catalytic reagents for organic synthesis . It plays a significant role in the fabrication of various shapes of carbon nanostructures .
Chemical Vapor Deposition (CVD)
The compound is used in the CVD process to form thin films . These films are used in thermal barrier coatings, anti-corrosion layers, high-k dielectric layers, anti-reflective coatings, dielectric capacitors, or for energy storage applications .
Crosslinking Agent for Gravure Inks
Orgatix ZC 150 serves as a crosslinking agent for gravure inks . This application enhances the durability and quality of the printed material .
Curing Catalyst for Urethane Resin
It is used as a curing catalyst for urethane resin . This accelerates the hardening process of the resin, improving its mechanical properties .
Catalyst for Silanol Condensation
Orgatix ZC 150 is used as a catalyst for silanol condensation . This process is crucial in the production of silicon-based polymers .
Formation of Zirconium Oxide Layers
The compound is used to form zirconium oxide layers on various materials . These layers can provide corrosion resistance, thermal insulation, and other beneficial properties .
Mechanism of Action
Target of Action
Zirconium acetylacetonate, also known as Orgatix ZC 150, is a coordination complex derived from the acetylacetonate anion and zirconium ions . This compound is widely used as a precursor for nanoparticle research, polymer science, and catalysis . Its primary targets are the molecules or structures it interacts with during these processes.
Mode of Action
The compound interacts with its targets through the formation of a six-membered chelate ring, typically binding both oxygen atoms to the metal . This interaction results in the formation of metal enolates, which are widely used as building blocks in modern organic synthesis . The compound’s reactivity and regio- and stereoselectivity have been attributed to aggregation .
Biochemical Pathways
The compound affects several biochemical pathways. For instance, in the synthesis of Zirconium-based nanomaterials, the compound undergoes thermal decomposition, forming important zirconium intermediates . These intermediates then participate in various reactions, leading to the formation of the desired nanomaterials .
Result of Action
The action of Zirconium acetylacetonate results in various molecular and cellular effects. For instance, it’s used as a sol-gel precursor to synthesize Zirconium-based nanomaterials with photocatalytic activity . It’s also used as an additive to improve the performance of the electron transport layer in perovskite solar cells .
Action Environment
The action, efficacy, and stability of Zirconium acetylacetonate can be influenced by environmental factors. For example, the choice of dilution gas significantly influences the temperature profile and residence times in the microreactor, affecting the thermal decomposition of the compound . Furthermore, the surface roughness of the nanostructure can influence the adhesion force and early attachment of Streptococcus mutans on the zirconia .
Safety and Hazards
Future Directions
The thermal decomposition of Zr(acac)4 is studied in a SiC-microreactor on the micro-second time scale . The ultimate goal of this effort is towards the development of catalysts that impart the selectivities, reactivity, and atom economy needed for cost-effective approaches to a variety of critical chemical transformations .
properties
IUPAC Name |
(Z)-4-oxopent-2-en-2-olate;zirconium(4+) | |
---|---|---|
Source | PubChem | |
URL | https://pubchem.ncbi.nlm.nih.gov | |
Description | Data deposited in or computed by PubChem | |
InChI |
InChI=1S/4C5H8O2.Zr/c4*1-4(6)3-5(2)7;/h4*3,6H,1-2H3;/q;;;;+4/p-4/b4*4-3-; | |
Source | PubChem | |
URL | https://pubchem.ncbi.nlm.nih.gov | |
Description | Data deposited in or computed by PubChem | |
InChI Key |
FPFOSIXCIBGKOH-MTOQALJVSA-J | |
Source | PubChem | |
URL | https://pubchem.ncbi.nlm.nih.gov | |
Description | Data deposited in or computed by PubChem | |
Canonical SMILES |
CC(=CC(=O)C)[O-].CC(=CC(=O)C)[O-].CC(=CC(=O)C)[O-].CC(=CC(=O)C)[O-].[Zr+4] | |
Source | PubChem | |
URL | https://pubchem.ncbi.nlm.nih.gov | |
Description | Data deposited in or computed by PubChem | |
Isomeric SMILES |
C/C(=C/C(=O)C)/[O-].C/C(=C/C(=O)C)/[O-].C/C(=C/C(=O)C)/[O-].C/C(=C/C(=O)C)/[O-].[Zr+4] | |
Source | PubChem | |
URL | https://pubchem.ncbi.nlm.nih.gov | |
Description | Data deposited in or computed by PubChem | |
Molecular Formula |
C20H28O8Zr | |
Source | PubChem | |
URL | https://pubchem.ncbi.nlm.nih.gov | |
Description | Data deposited in or computed by PubChem | |
DSSTOX Substance ID |
DTXSID00904296 | |
Record name | Tetrakis(pentane-2,4-dionato-O,O')zirconium | |
Source | EPA DSSTox | |
URL | https://comptox.epa.gov/dashboard/DTXSID00904296 | |
Description | DSSTox provides a high quality public chemistry resource for supporting improved predictive toxicology. | |
Molecular Weight |
487.7 g/mol | |
Source | PubChem | |
URL | https://pubchem.ncbi.nlm.nih.gov | |
Description | Data deposited in or computed by PubChem | |
Physical Description |
Off-white powder; [MSDSonline] | |
Record name | Zirconium acetylacetonate | |
Source | Haz-Map, Information on Hazardous Chemicals and Occupational Diseases | |
URL | https://haz-map.com/Agents/8461 | |
Description | Haz-Map® is an occupational health database designed for health and safety professionals and for consumers seeking information about the adverse effects of workplace exposures to chemical and biological agents. | |
Explanation | Copyright (c) 2022 Haz-Map(R). All rights reserved. Unless otherwise indicated, all materials from Haz-Map are copyrighted by Haz-Map(R). No part of these materials, either text or image may be used for any purpose other than for personal use. Therefore, reproduction, modification, storage in a retrieval system or retransmission, in any form or by any means, electronic, mechanical or otherwise, for reasons other than personal use, is strictly prohibited without prior written permission. | |
CAS RN |
17501-44-9 | |
Record name | Zirconium acetylacetonate | |
Source | ChemIDplus | |
URL | https://pubchem.ncbi.nlm.nih.gov/substance/?source=chemidplus&sourceid=0017501449 | |
Description | ChemIDplus is a free, web search system that provides access to the structure and nomenclature authority files used for the identification of chemical substances cited in National Library of Medicine (NLM) databases, including the TOXNET system. | |
Record name | Zirconium, tetrakis(2,4-pentanedionato-.kappa.O2,.kappa.O4)-, (SA-8-11''11''1'1'''1'1''')- | |
Source | EPA Chemicals under the TSCA | |
URL | https://www.epa.gov/chemicals-under-tsca | |
Description | EPA Chemicals under the Toxic Substances Control Act (TSCA) collection contains information on chemicals and their regulations under TSCA, including non-confidential content from the TSCA Chemical Substance Inventory and Chemical Data Reporting. | |
Record name | Tetrakis(pentane-2,4-dionato-O,O')zirconium | |
Source | EPA DSSTox | |
URL | https://comptox.epa.gov/dashboard/DTXSID00904296 | |
Description | DSSTox provides a high quality public chemistry resource for supporting improved predictive toxicology. | |
Record name | Tetrakis(pentane-2,4-dionato-O,O')zirconium | |
Source | European Chemicals Agency (ECHA) | |
URL | https://echa.europa.eu/substance-information/-/substanceinfo/100.037.721 | |
Description | The European Chemicals Agency (ECHA) is an agency of the European Union which is the driving force among regulatory authorities in implementing the EU's groundbreaking chemicals legislation for the benefit of human health and the environment as well as for innovation and competitiveness. | |
Explanation | Use of the information, documents and data from the ECHA website is subject to the terms and conditions of this Legal Notice, and subject to other binding limitations provided for under applicable law, the information, documents and data made available on the ECHA website may be reproduced, distributed and/or used, totally or in part, for non-commercial purposes provided that ECHA is acknowledged as the source: "Source: European Chemicals Agency, http://echa.europa.eu/". Such acknowledgement must be included in each copy of the material. ECHA permits and encourages organisations and individuals to create links to the ECHA website under the following cumulative conditions: Links can only be made to webpages that provide a link to the Legal Notice page. | |
Record name | ZIRCONIUM ACETYLACETONATE | |
Source | FDA Global Substance Registration System (GSRS) | |
URL | https://gsrs.ncats.nih.gov/ginas/app/beta/substances/15NW5BA32K | |
Description | The FDA Global Substance Registration System (GSRS) enables the efficient and accurate exchange of information on what substances are in regulated products. Instead of relying on names, which vary across regulatory domains, countries, and regions, the GSRS knowledge base makes it possible for substances to be defined by standardized, scientific descriptions. | |
Explanation | Unless otherwise noted, the contents of the FDA website (www.fda.gov), both text and graphics, are not copyrighted. They are in the public domain and may be republished, reprinted and otherwise used freely by anyone without the need to obtain permission from FDA. Credit to the U.S. Food and Drug Administration as the source is appreciated but not required. | |
Retrosynthesis Analysis
AI-Powered Synthesis Planning: Our tool employs the Template_relevance Pistachio, Template_relevance Bkms_metabolic, Template_relevance Pistachio_ringbreaker, Template_relevance Reaxys, Template_relevance Reaxys_biocatalysis model, leveraging a vast database of chemical reactions to predict feasible synthetic routes.
One-Step Synthesis Focus: Specifically designed for one-step synthesis, it provides concise and direct routes for your target compounds, streamlining the synthesis process.
Accurate Predictions: Utilizing the extensive PISTACHIO, BKMS_METABOLIC, PISTACHIO_RINGBREAKER, REAXYS, REAXYS_BIOCATALYSIS database, our tool offers high-accuracy predictions, reflecting the latest in chemical research and data.
Strategy Settings
Precursor scoring | Relevance Heuristic |
---|---|
Min. plausibility | 0.01 |
Model | Template_relevance |
Template Set | Pistachio/Bkms_metabolic/Pistachio_ringbreaker/Reaxys/Reaxys_biocatalysis |
Top-N result to add to graph | 6 |
Feasible Synthetic Routes
Disclaimer and Information on In-Vitro Research Products
Please be aware that all articles and product information presented on BenchChem are intended solely for informational purposes. The products available for purchase on BenchChem are specifically designed for in-vitro studies, which are conducted outside of living organisms. In-vitro studies, derived from the Latin term "in glass," involve experiments performed in controlled laboratory settings using cells or tissues. It is important to note that these products are not categorized as medicines or drugs, and they have not received approval from the FDA for the prevention, treatment, or cure of any medical condition, ailment, or disease. We must emphasize that any form of bodily introduction of these products into humans or animals is strictly prohibited by law. It is essential to adhere to these guidelines to ensure compliance with legal and ethical standards in research and experimentation.