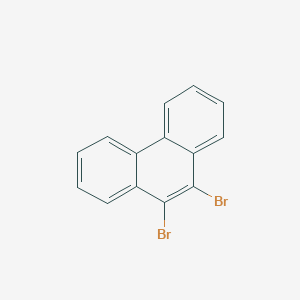
9,10-Dibromophenanthrene
Overview
Description
9,10-Dibromophenanthrene is an organic compound with the molecular formula C14H8Br2. It is a derivative of phenanthrene, where two bromine atoms are substituted at the 9th and 10th positions of the phenanthrene ring system. This compound is known for its planar structure and crystallographic twofold symmetry .
Preparation Methods
Synthetic Routes and Reaction Conditions: 9,10-Dibromophenanthrene can be synthesized through the bromination of phenanthrene. The reaction typically involves the use of bromine in the presence of a solvent such as carbon tetrachloride (CCl4) at room temperature. The reaction proceeds as follows: [ \text{Phenanthrene} + \text{Br}_2 \rightarrow \text{this compound} ]
Industrial Production Methods: In an industrial setting, the synthesis of this compound may involve the use of bromine in the presence of a catalyst such as iron(III) bromide (FeBr3) to enhance the reaction rate and yield .
Types of Reactions:
Oxidation: this compound can undergo oxidation reactions to form phenanthraquinone derivatives.
Reduction: Reduction of this compound can lead to the formation of 9,10-dihydrophenanthrene.
Substitution: The bromine atoms in this compound can be substituted with other functional groups through nucleophilic substitution reactions.
Common Reagents and Conditions:
Oxidation: Potassium permanganate (KMnO4) in acidic conditions.
Reduction: Sodium (Na) in isopentanol.
Substitution: Nucleophiles such as amines or thiols in the presence of a base.
Major Products:
Oxidation: Phenanthraquinone derivatives.
Reduction: 9,10-Dihydrophenanthrene.
Substitution: Various substituted phenanthrene derivatives depending on the nucleophile used.
Scientific Research Applications
9,10-Dibromophenanthrene has several applications in scientific research:
Chemistry: It is used as a precursor in the synthesis of more complex organic molecules and polymers.
Biology: It serves as a model compound for studying the interactions of polycyclic aromatic hydrocarbons with biological systems.
Medicine: Research is ongoing to explore its potential use in drug development and as a probe for studying biological processes.
Industry: It is used in the production of materials with specific electronic and optical properties.
Mechanism of Action
The mechanism of action of 9,10-Dibromophenanthrene involves its interaction with various molecular targets. The bromine atoms at the 9th and 10th positions make the compound highly reactive, allowing it to participate in a variety of chemical reactions. These interactions can lead to the formation of reactive intermediates that can further react with other molecules, influencing various chemical pathways .
Comparison with Similar Compounds
9,10-Dibromoanthracene: Similar in structure but with an anthracene core instead of phenanthrene.
2,7-Dibromophenanthrene-9,10-dione: Contains additional functional groups that make it useful in different applications.
Uniqueness: 9,10-Dibromophenanthrene is unique due to its specific substitution pattern on the phenanthrene ring, which imparts distinct chemical reactivity and physical properties. This makes it particularly useful in the synthesis of specialized organic compounds and materials .
Properties
IUPAC Name |
9,10-dibromophenanthrene | |
---|---|---|
Source | PubChem | |
URL | https://pubchem.ncbi.nlm.nih.gov | |
Description | Data deposited in or computed by PubChem | |
InChI |
InChI=1S/C14H8Br2/c15-13-11-7-3-1-5-9(11)10-6-2-4-8-12(10)14(13)16/h1-8H | |
Source | PubChem | |
URL | https://pubchem.ncbi.nlm.nih.gov | |
Description | Data deposited in or computed by PubChem | |
InChI Key |
WZVDACBKJRRYBN-UHFFFAOYSA-N | |
Source | PubChem | |
URL | https://pubchem.ncbi.nlm.nih.gov | |
Description | Data deposited in or computed by PubChem | |
Canonical SMILES |
C1=CC=C2C(=C1)C3=CC=CC=C3C(=C2Br)Br | |
Source | PubChem | |
URL | https://pubchem.ncbi.nlm.nih.gov | |
Description | Data deposited in or computed by PubChem | |
Molecular Formula |
C14H8Br2 | |
Source | PubChem | |
URL | https://pubchem.ncbi.nlm.nih.gov | |
Description | Data deposited in or computed by PubChem | |
DSSTOX Substance ID |
DTXSID10399891 | |
Record name | 9,10-dibromophenanthrene | |
Source | EPA DSSTox | |
URL | https://comptox.epa.gov/dashboard/DTXSID10399891 | |
Description | DSSTox provides a high quality public chemistry resource for supporting improved predictive toxicology. | |
Molecular Weight |
336.02 g/mol | |
Source | PubChem | |
URL | https://pubchem.ncbi.nlm.nih.gov | |
Description | Data deposited in or computed by PubChem | |
CAS No. |
15810-15-8 | |
Record name | 9,10-dibromophenanthrene | |
Source | EPA DSSTox | |
URL | https://comptox.epa.gov/dashboard/DTXSID10399891 | |
Description | DSSTox provides a high quality public chemistry resource for supporting improved predictive toxicology. | |
Record name | 9,10-DIBROMOPHENANTHRENE | |
Source | European Chemicals Agency (ECHA) | |
URL | https://echa.europa.eu/information-on-chemicals | |
Description | The European Chemicals Agency (ECHA) is an agency of the European Union which is the driving force among regulatory authorities in implementing the EU's groundbreaking chemicals legislation for the benefit of human health and the environment as well as for innovation and competitiveness. | |
Explanation | Use of the information, documents and data from the ECHA website is subject to the terms and conditions of this Legal Notice, and subject to other binding limitations provided for under applicable law, the information, documents and data made available on the ECHA website may be reproduced, distributed and/or used, totally or in part, for non-commercial purposes provided that ECHA is acknowledged as the source: "Source: European Chemicals Agency, http://echa.europa.eu/". Such acknowledgement must be included in each copy of the material. ECHA permits and encourages organisations and individuals to create links to the ECHA website under the following cumulative conditions: Links can only be made to webpages that provide a link to the Legal Notice page. | |
Retrosynthesis Analysis
AI-Powered Synthesis Planning: Our tool employs the Template_relevance Pistachio, Template_relevance Bkms_metabolic, Template_relevance Pistachio_ringbreaker, Template_relevance Reaxys, Template_relevance Reaxys_biocatalysis model, leveraging a vast database of chemical reactions to predict feasible synthetic routes.
One-Step Synthesis Focus: Specifically designed for one-step synthesis, it provides concise and direct routes for your target compounds, streamlining the synthesis process.
Accurate Predictions: Utilizing the extensive PISTACHIO, BKMS_METABOLIC, PISTACHIO_RINGBREAKER, REAXYS, REAXYS_BIOCATALYSIS database, our tool offers high-accuracy predictions, reflecting the latest in chemical research and data.
Strategy Settings
Precursor scoring | Relevance Heuristic |
---|---|
Min. plausibility | 0.01 |
Model | Template_relevance |
Template Set | Pistachio/Bkms_metabolic/Pistachio_ringbreaker/Reaxys/Reaxys_biocatalysis |
Top-N result to add to graph | 6 |
Feasible Synthetic Routes
Q1: What is the molecular structure and some key physical properties of 9,10-Dibromophenanthrene?
A1: this compound (C14H8Br2) is a planar molecule with a crystallographic twofold (C2) symmetry []. This means the molecule can be bisected into two identical halves. The crystal structure reveals that the molecules engage in face-to-face slipped antiparallel π–π stacking interactions along the c axis []. This type of interaction is common in aromatic systems and contributes to the molecule's overall stability in its solid state.
Q2: How does the presence of bromine atoms in this compound affect its vapor pressure compared to its parent compound, phenanthrene?
A2: Research indicates that halogen substitution, such as the bromine atoms in this compound, generally leads to a decrease in vapor pressure compared to the parent compound, phenanthrene []. This decrease is attributed to the increased molecular weight and stronger intermolecular forces (like the π–π stacking mentioned earlier) caused by the presence of the halogen atoms. Interestingly, while halogenation usually decreases vapor pressure, it doesn't always lead to a higher enthalpy of sublimation [].
Disclaimer and Information on In-Vitro Research Products
Please be aware that all articles and product information presented on BenchChem are intended solely for informational purposes. The products available for purchase on BenchChem are specifically designed for in-vitro studies, which are conducted outside of living organisms. In-vitro studies, derived from the Latin term "in glass," involve experiments performed in controlled laboratory settings using cells or tissues. It is important to note that these products are not categorized as medicines or drugs, and they have not received approval from the FDA for the prevention, treatment, or cure of any medical condition, ailment, or disease. We must emphasize that any form of bodily introduction of these products into humans or animals is strictly prohibited by law. It is essential to adhere to these guidelines to ensure compliance with legal and ethical standards in research and experimentation.