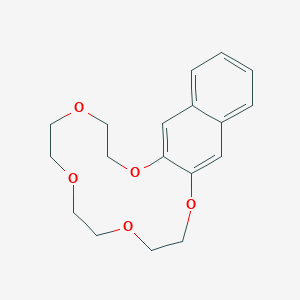
2,3-Naphtho-15-crown-5
Overview
Description
2,3-Naphtho-15-crown-5: is a type of crown ether, which is a class of cyclic chemical compounds that consist of a ring containing several ether groups. Crown ethers are known for their ability to form stable complexes with metal cations due to their unique structure. The “15-crown-5” part of the name indicates that the compound has a ring consisting of fifteen atoms, including five oxygen atoms. The “2,3-naphtho” part indicates that the crown ether is fused with a naphthalene ring at the 2 and 3 positions. This compound is of significant interest due to its ability to selectively bind to specific metal cations, making it useful in various chemical and industrial applications .
Mechanism of Action
Target of Action
The primary targets of 2,3-Naphtho-15-crown-5 (N15C5) are metal cations . Crown ethers, such as N15C5, have been the subject of great interest due to their selective capability to bind to metal cations . The use of a constant crown ether, such as N15C5, and varied metal cations (Li+, Na+, K+, Be2+, Mg2+, Ca2+, Co2+, Ni2+, Cu2+) makes it possible to determine the contributions of the metal cations to nonlinear optical (NLO) responses .
Mode of Action
N15C5 interacts with its targets, the metal cations, through a process known as cation-complexing . This interaction results in changes in the first hyperpolarizability of the compound . The dependency of the first hyperpolarizability relies on the metal cation, especially for transition metals .
Biochemical Pathways
The biochemical pathways affected by N15C5 involve the interactions of the compound with metal cations. The compound’s interaction with these cations leads to changes in their nonlinear optical (NLO) responses . These changes can be used to design an appropriate NLO-based cation detector .
Result of Action
The interaction of N15C5 with metal cations results in changes in the first hyperpolarizability of the compound . The decrease of the first hyperpolarizabilities for alkali metal cation derivatives is due to their relatively low oscillator strengths, whereas the significant increase of the first hyperpolarizabilities for transition metal cation derivatives can be further illustrated by their low transition energies, large amplitudes, and separate distributions of first hyperpolarizability density . Thus, the alkali metal and transition metal cations are distinguishable, and the transition metal cations are easier to detect by utilizing the variations in NLO responses .
Biochemical Analysis
Biochemical Properties
The biochemical properties of 2,3-Naphtho-15-crown-5 are largely defined by its ability to bind to various metal cations. This includes alkali metal cations such as lithium (Li+), sodium (Na+), and potassium (K+), as well as transition metal cations like beryllium (Be2+), magnesium (Mg2+), calcium (Ca2+), cobalt (Co2+), nickel (Ni2+), and copper (Cu2+) . The binding of these cations to this compound can influence the compound’s nonlinear optical (NLO) responses, which can be utilized in the design of NLO-based cation detectors .
Molecular Mechanism
The molecular mechanism of this compound is primarily based on its ability to bind to various metal cations. The binding of these cations can influence the compound’s first hyperpolarizability, a property related to its NLO responses . The dependency of the first hyperpolarizability on the metal cation is especially notable for transition metals .
Preparation Methods
Synthetic Routes and Reaction Conditions: The synthesis of 2,3-Naphtho-15-crown-5 typically involves the reaction of a naphthalene derivative with a suitable diol under acidic conditions to form the crown ether ring. One common method involves the use of 2,3-dihydroxynaphthalene and tetraethylene glycol in the presence of a strong acid catalyst such as sulfuric acid. The reaction proceeds through a series of condensation steps to form the desired crown ether .
Industrial Production Methods: In an industrial setting, the production of this compound may involve similar synthetic routes but on a larger scale. The reaction conditions are optimized to ensure high yield and purity of the product. This may include the use of continuous flow reactors and advanced purification techniques such as distillation and crystallization .
Chemical Reactions Analysis
Types of Reactions: 2,3-Naphtho-15-crown-5 undergoes various types of chemical reactions, including:
Complexation Reactions: It forms stable complexes with metal cations such as sodium, potassium, and calcium.
Substitution Reactions: The naphthalene ring can undergo electrophilic substitution reactions, such as nitration and sulfonation, due to the presence of electron-donating ether groups.
Common Reagents and Conditions:
Complexation Reactions: Typically involve the use of metal salts such as sodium chloride or potassium nitrate in an aqueous or organic solvent.
Substitution Reactions: Common reagents include nitric acid for nitration and sulfuric acid for sulfonation.
Major Products Formed:
Complexation Reactions: The major products are metal-crown ether complexes, which are often used in analytical chemistry and separation processes.
Substitution Reactions: The major products include nitro and sulfonated derivatives of this compound.
Scientific Research Applications
2,3-Naphtho-15-crown-5 has a wide range of applications in scientific research, including:
Comparison with Similar Compounds
15-Crown-5: A simpler crown ether with a similar ring structure but without the naphthalene fusion.
Dibenzo-18-crown-6: A crown ether with two benzene rings fused to the crown ether ring, providing additional stability and selectivity for certain metal cations.
Uniqueness of 2,3-Naphtho-15-crown-5: The unique feature of this compound is the presence of the naphthalene ring fused to the crown ether ring. This fusion enhances the stability and selectivity of the metal-cation complexes formed, making it particularly useful in applications requiring high specificity .
Properties
IUPAC Name |
2,5,8,11,14-pentaoxatricyclo[13.8.0.017,22]tricosa-1(23),15,17,19,21-pentaene | |
---|---|---|
Source | PubChem | |
URL | https://pubchem.ncbi.nlm.nih.gov | |
Description | Data deposited in or computed by PubChem | |
InChI |
InChI=1S/C18H22O5/c1-2-4-16-14-18-17(13-15(16)3-1)22-11-9-20-7-5-19-6-8-21-10-12-23-18/h1-4,13-14H,5-12H2 | |
Source | PubChem | |
URL | https://pubchem.ncbi.nlm.nih.gov | |
Description | Data deposited in or computed by PubChem | |
InChI Key |
BDTDDXDRCOLVNJ-UHFFFAOYSA-N | |
Source | PubChem | |
URL | https://pubchem.ncbi.nlm.nih.gov | |
Description | Data deposited in or computed by PubChem | |
Canonical SMILES |
C1COCCOC2=CC3=CC=CC=C3C=C2OCCOCCO1 | |
Source | PubChem | |
URL | https://pubchem.ncbi.nlm.nih.gov | |
Description | Data deposited in or computed by PubChem | |
Molecular Formula |
C18H22O5 | |
Source | PubChem | |
URL | https://pubchem.ncbi.nlm.nih.gov | |
Description | Data deposited in or computed by PubChem | |
DSSTOX Substance ID |
DTXSID30375051 | |
Record name | 2,3-Naphtho-15-crown-5 | |
Source | EPA DSSTox | |
URL | https://comptox.epa.gov/dashboard/DTXSID30375051 | |
Description | DSSTox provides a high quality public chemistry resource for supporting improved predictive toxicology. | |
Molecular Weight |
318.4 g/mol | |
Source | PubChem | |
URL | https://pubchem.ncbi.nlm.nih.gov | |
Description | Data deposited in or computed by PubChem | |
CAS No. |
17454-47-6 | |
Record name | 2,3-Naphtho-15-crown-5 | |
Source | EPA DSSTox | |
URL | https://comptox.epa.gov/dashboard/DTXSID30375051 | |
Description | DSSTox provides a high quality public chemistry resource for supporting improved predictive toxicology. | |
Record name | 2,3-Naphtho-15-crown-5 | |
Source | European Chemicals Agency (ECHA) | |
URL | https://echa.europa.eu/information-on-chemicals | |
Description | The European Chemicals Agency (ECHA) is an agency of the European Union which is the driving force among regulatory authorities in implementing the EU's groundbreaking chemicals legislation for the benefit of human health and the environment as well as for innovation and competitiveness. | |
Explanation | Use of the information, documents and data from the ECHA website is subject to the terms and conditions of this Legal Notice, and subject to other binding limitations provided for under applicable law, the information, documents and data made available on the ECHA website may be reproduced, distributed and/or used, totally or in part, for non-commercial purposes provided that ECHA is acknowledged as the source: "Source: European Chemicals Agency, http://echa.europa.eu/". Such acknowledgement must be included in each copy of the material. ECHA permits and encourages organisations and individuals to create links to the ECHA website under the following cumulative conditions: Links can only be made to webpages that provide a link to the Legal Notice page. | |
Retrosynthesis Analysis
AI-Powered Synthesis Planning: Our tool employs the Template_relevance Pistachio, Template_relevance Bkms_metabolic, Template_relevance Pistachio_ringbreaker, Template_relevance Reaxys, Template_relevance Reaxys_biocatalysis model, leveraging a vast database of chemical reactions to predict feasible synthetic routes.
One-Step Synthesis Focus: Specifically designed for one-step synthesis, it provides concise and direct routes for your target compounds, streamlining the synthesis process.
Accurate Predictions: Utilizing the extensive PISTACHIO, BKMS_METABOLIC, PISTACHIO_RINGBREAKER, REAXYS, REAXYS_BIOCATALYSIS database, our tool offers high-accuracy predictions, reflecting the latest in chemical research and data.
Strategy Settings
Precursor scoring | Relevance Heuristic |
---|---|
Min. plausibility | 0.01 |
Model | Template_relevance |
Template Set | Pistachio/Bkms_metabolic/Pistachio_ringbreaker/Reaxys/Reaxys_biocatalysis |
Top-N result to add to graph | 6 |
Feasible Synthetic Routes
Disclaimer and Information on In-Vitro Research Products
Please be aware that all articles and product information presented on BenchChem are intended solely for informational purposes. The products available for purchase on BenchChem are specifically designed for in-vitro studies, which are conducted outside of living organisms. In-vitro studies, derived from the Latin term "in glass," involve experiments performed in controlled laboratory settings using cells or tissues. It is important to note that these products are not categorized as medicines or drugs, and they have not received approval from the FDA for the prevention, treatment, or cure of any medical condition, ailment, or disease. We must emphasize that any form of bodily introduction of these products into humans or animals is strictly prohibited by law. It is essential to adhere to these guidelines to ensure compliance with legal and ethical standards in research and experimentation.