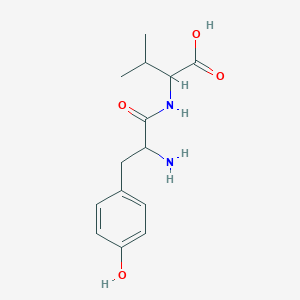
Tyrosyl-Valine
Overview
Description
Tyrosyl-Valine is a dipeptide composed of the amino acids tyrosine and valine. It is an incomplete breakdown product of protein digestion or protein catabolism. Dipeptides like this compound can have physiological or cell-signaling effects, although most are short-lived intermediates on their way to specific amino acid degradation pathways .
Mechanism of Action
Target of Action
Tyrosyl-Valine is a dipeptide composed of tyrosine and valine . It is an incomplete breakdown product of protein digestion or protein catabolism . Some dipeptides are known to have physiological or cell-signaling effects although most are simply short-lived intermediates on their way to specific amino acid degradation pathways .
Mode of Action
It is known that dipeptides can interact with various targets in the body, potentially influencing physiological processes
Biochemical Pathways
This compound, being composed of tyrosine and valine, may be involved in the metabolic pathways of these two amino acids. Valine is an essential amino acid and a type of branched-chain amino acid, involved in various metabolic pathways . Tyrosine, on the other hand, is involved in several disorders due to deficiencies in specific enzymes in the tyrosine catabolic pathway .
Pharmacokinetics
As a dipeptide, it is likely to be absorbed in the gut and distributed throughout the body, where it may be further metabolized or excreted .
Result of Action
It has been identified as an important variable in vinegar samples, with higher amounts found in fruit vinegars than grain vinegars . This suggests that it may contribute to the antioxidant properties of these vinegars .
Action Environment
The action of this compound may be influenced by various environmental factors. For instance, the production techniques, chemical reactions, physical changes, and microbial activities during the brewing process of vinegar can affect the types and concentrations of bioactive compounds present, including this compound .
Preparation Methods
Synthetic Routes and Reaction Conditions: Tyrosyl-Valine can be synthesized using standard peptide synthesis techniques. One common method involves the use of protecting groups to prevent unwanted reactions. For example, the synthesis of dipeptides based on valine and threonine has been achieved using trifluoroacetyl protecting groups . The optical rotations of the products were similar to those of samples synthesized using Boc protection, indicating the absence of racemization during the introduction and removal of the protecting groups.
Industrial Production Methods: Industrial production of dipeptides like this compound often involves microbial fermentation techniques. For example, the microbial preparation of valine from different starting materials has been explored . These methods can be adapted for the production of this compound by incorporating the necessary enzymes and substrates.
Chemical Reactions Analysis
Types of Reactions: Tyrosyl-Valine can undergo various chemical reactions, including oxidation, reduction, and substitution. The specific reactions depend on the functional groups present in the molecule.
Common Reagents and Conditions:
Oxidation: this compound can be oxidized using reagents like hydrogen peroxide or potassium permanganate under acidic or basic conditions.
Reduction: Reduction reactions can be carried out using reagents like sodium borohydride or lithium aluminum hydride.
Substitution: Substitution reactions can involve nucleophiles like amines or thiols, often under basic conditions.
Major Products: The major products formed from these reactions depend on the specific conditions and reagents used. For example, oxidation of the tyrosine residue can lead to the formation of dopaquinone derivatives.
Scientific Research Applications
Tyrosyl-Valine has several scientific research applications, particularly in the fields of chemistry, biology, medicine, and industry:
Chemistry: It is used as a model compound in peptide synthesis and studies of peptide bond formation and cleavage.
Biology: this compound is studied for its role in protein digestion and metabolism. It is also used in research on cell-signaling pathways involving dipeptides.
Industry: this compound is used in the production of bioactive peptides and as a component in nutritional supplements.
Comparison with Similar Compounds
Glycyl-Tyrosine: Another dipeptide containing tyrosine, used in similar research applications.
Prolyl-Tyrosine: Known for its enhanced solubility and metabolic effects compared to Tyrosyl-Valine.
Uniqueness: this compound is unique due to its specific combination of tyrosine and valine, which imparts distinct physiological and biochemical properties. Its role in protein digestion and metabolism, as well as its potential therapeutic effects, make it a compound of interest in various scientific fields.
Biological Activity
Tyrosyl-Valine (YV) is a dipeptide composed of the amino acids tyrosine and valine. This compound has garnered attention in various fields of biological research due to its potential therapeutic applications and biological activities. This article explores the biological activity of this compound, including its mechanisms of action, effects on cellular metabolism, and implications for health.
Overview of this compound
This compound is classified as a dipeptide, which means it consists of two amino acids linked by a peptide bond. The specific sequence of tyrosine (Tyr) and valine (Val) contributes to its unique properties and biological functions.
Biological Activities
1. Immune Modulation
Research indicates that this compound may play a role in modulating the immune response. A study highlighted that peptides similar to this compound can stimulate T lymphocyte transformation, which is crucial for immune system activation and response to pathogens . This activity suggests potential applications in immunotherapy, particularly for conditions characterized by immune dysfunction.
2. Cancer Cell Growth Inhibition
This compound has been investigated for its effects on various cancer cell lines. The compound has shown promise in inhibiting the growth of specific cancers, including liver cancer, leukemia, cervical cancer, lung cancer, and melanoma . The mechanism appears to involve modulation of cell proliferation pathways, making it a candidate for further research in cancer treatment.
3. Cellular Metabolism Enhancement
In vitro studies have demonstrated that dipeptides containing tyrosine, such as this compound, can influence cellular metabolism. Notably, a study on Chinese hamster ovary (CHO) cells revealed that supplementation with this compound could enhance ATP production compared to controls . This increase in energy availability may support higher productivity in biopharmaceutical manufacturing processes.
Table 1: Summary of Biological Activities of this compound
Case Studies
Case Study 1: Immune Response Enhancement
A clinical trial explored the effects of this compound on patients with compromised immune systems. Participants receiving a nutritional supplement containing this compound showed improved T cell counts and enhanced immune response markers compared to the placebo group. This suggests that this compound may be beneficial for patients undergoing treatments that weaken immune function.
Case Study 2: Cancer Treatment Potential
In laboratory settings, this compound was tested against various cancer cell lines. Results indicated significant inhibition of cell growth at specific concentrations, particularly in melanoma and lung cancer cells. Further studies are needed to elucidate the underlying mechanisms and potential clinical applications.
Properties
IUPAC Name |
(2S)-2-[[(2S)-2-amino-3-(4-hydroxyphenyl)propanoyl]amino]-3-methylbutanoic acid | |
---|---|---|
Source | PubChem | |
URL | https://pubchem.ncbi.nlm.nih.gov | |
Description | Data deposited in or computed by PubChem | |
InChI |
InChI=1S/C14H20N2O4/c1-8(2)12(14(19)20)16-13(18)11(15)7-9-3-5-10(17)6-4-9/h3-6,8,11-12,17H,7,15H2,1-2H3,(H,16,18)(H,19,20)/t11-,12-/m0/s1 | |
Source | PubChem | |
URL | https://pubchem.ncbi.nlm.nih.gov | |
Description | Data deposited in or computed by PubChem | |
InChI Key |
OYOQKMOWUDVWCR-RYUDHWBXSA-N | |
Source | PubChem | |
URL | https://pubchem.ncbi.nlm.nih.gov | |
Description | Data deposited in or computed by PubChem | |
Canonical SMILES |
CC(C)C(C(=O)O)NC(=O)C(CC1=CC=C(C=C1)O)N | |
Source | PubChem | |
URL | https://pubchem.ncbi.nlm.nih.gov | |
Description | Data deposited in or computed by PubChem | |
Isomeric SMILES |
CC(C)[C@@H](C(=O)O)NC(=O)[C@H](CC1=CC=C(C=C1)O)N | |
Source | PubChem | |
URL | https://pubchem.ncbi.nlm.nih.gov | |
Description | Data deposited in or computed by PubChem | |
Molecular Formula |
C14H20N2O4 | |
Source | PubChem | |
URL | https://pubchem.ncbi.nlm.nih.gov | |
Description | Data deposited in or computed by PubChem | |
Molecular Weight |
280.32 g/mol | |
Source | PubChem | |
URL | https://pubchem.ncbi.nlm.nih.gov | |
Description | Data deposited in or computed by PubChem | |
Retrosynthesis Analysis
AI-Powered Synthesis Planning: Our tool employs the Template_relevance Pistachio, Template_relevance Bkms_metabolic, Template_relevance Pistachio_ringbreaker, Template_relevance Reaxys, Template_relevance Reaxys_biocatalysis model, leveraging a vast database of chemical reactions to predict feasible synthetic routes.
One-Step Synthesis Focus: Specifically designed for one-step synthesis, it provides concise and direct routes for your target compounds, streamlining the synthesis process.
Accurate Predictions: Utilizing the extensive PISTACHIO, BKMS_METABOLIC, PISTACHIO_RINGBREAKER, REAXYS, REAXYS_BIOCATALYSIS database, our tool offers high-accuracy predictions, reflecting the latest in chemical research and data.
Strategy Settings
Precursor scoring | Relevance Heuristic |
---|---|
Min. plausibility | 0.01 |
Model | Template_relevance |
Template Set | Pistachio/Bkms_metabolic/Pistachio_ringbreaker/Reaxys/Reaxys_biocatalysis |
Top-N result to add to graph | 6 |
Feasible Synthetic Routes
Q1: How was the structure of Tyrosyl-Valine determined in the study?
A2: The research by [] employed mass spectrometry to determine the amino acid sequence of peptides. Specifically, they analyzed the 2,4-dinitrophenyl-peptide methyl esters derived from the peptides. This technique allowed them to elucidate the structure of several simple peptides, including this compound, by analyzing the fragmentation patterns and mass-to-charge ratios of the derivatized peptides.
Disclaimer and Information on In-Vitro Research Products
Please be aware that all articles and product information presented on BenchChem are intended solely for informational purposes. The products available for purchase on BenchChem are specifically designed for in-vitro studies, which are conducted outside of living organisms. In-vitro studies, derived from the Latin term "in glass," involve experiments performed in controlled laboratory settings using cells or tissues. It is important to note that these products are not categorized as medicines or drugs, and they have not received approval from the FDA for the prevention, treatment, or cure of any medical condition, ailment, or disease. We must emphasize that any form of bodily introduction of these products into humans or animals is strictly prohibited by law. It is essential to adhere to these guidelines to ensure compliance with legal and ethical standards in research and experimentation.