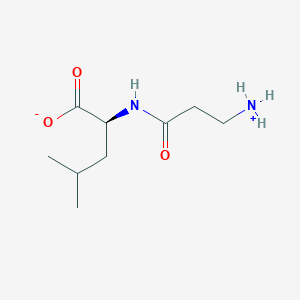
H-Beta-Ala-Leu-OH
- Click on QUICK INQUIRY to receive a quote from our team of experts.
- With the quality product at a COMPETITIVE price, you can focus more on your research.
Overview
Description
H-Beta-Ala-Leu-OH, also known as β-Alanyl-L-leucine, is a dipeptide composed of β-alanine and L-leucine. This compound is of interest due to its potential biological activities and applications in various fields, including chemistry, biology, and medicine.
Preparation Methods
Synthetic Routes and Reaction Conditions
The synthesis of H-Beta-Ala-Leu-OH typically involves the coupling of β-alanine and L-leucine. One common method is the use of peptide coupling reagents such as dicyclohexylcarbodiimide (DCC) and N-hydroxysuccinimide (NHS) to facilitate the formation of the peptide bond between the carboxyl group of β-alanine and the amino group of L-leucine. The reaction is usually carried out in an organic solvent like dimethylformamide (DMF) under mild conditions.
Industrial Production Methods
In an industrial setting, the production of this compound can be scaled up using automated peptide synthesizers. These machines allow for the precise control of reaction conditions and the efficient coupling of amino acids. The use of solid-phase peptide synthesis (SPPS) is also common, where the peptide is assembled on a solid resin support, allowing for easy purification and isolation of the final product.
Chemical Reactions Analysis
Types of Reactions
H-Beta-Ala-Leu-OH can undergo various chemical reactions, including:
Oxidation: The amino acid residues in the dipeptide can be oxidized under specific conditions, leading to the formation of oxidized derivatives.
Reduction: The compound can be reduced to form reduced derivatives, although this is less common.
Substitution: The amino and carboxyl groups in the dipeptide can participate in substitution reactions, leading to the formation of modified peptides.
Common Reagents and Conditions
Oxidation: Common oxidizing agents include hydrogen peroxide (H₂O₂) and potassium permanganate (KMnO₄).
Reduction: Reducing agents such as sodium borohydride (NaBH₄) can be used.
Substitution: Reagents like acyl chlorides and anhydrides can be used for acylation reactions.
Major Products Formed
The major products formed from these reactions depend on the specific conditions and reagents used. For example, oxidation can lead to the formation of oxidized peptides, while substitution reactions can yield acylated derivatives.
Scientific Research Applications
H-Beta-Ala-Leu-OH has several scientific research applications:
Chemistry: It is used as a model compound in peptide synthesis studies and in the development of new peptide coupling reagents.
Biology: The compound is studied for its potential role in modulating biological processes, such as enzyme activity and receptor binding.
Medicine: Research is ongoing to explore its potential therapeutic applications, including its use as a bioactive peptide in drug development.
Industry: this compound is used in the production of peptide-based materials and as a component in various biochemical assays.
Mechanism of Action
The mechanism of action of H-Beta-Ala-Leu-OH involves its interaction with specific molecular targets, such as enzymes and receptors. The dipeptide can bind to these targets and modulate their activity, leading to various biological effects. For example, it may inhibit or activate enzymes involved in metabolic pathways or bind to receptors and influence signal transduction processes.
Comparison with Similar Compounds
Similar Compounds
β-Alanyl-L-isoleucine: Similar in structure but contains isoleucine instead of leucine.
β-Alanyl-L-valine: Contains valine instead of leucine.
Carnosine (β-Alanyl-L-histidine): A well-known dipeptide with antioxidant properties.
Uniqueness
H-Beta-Ala-Leu-OH is unique due to its specific combination of β-alanine and L-leucine, which imparts distinct biological activities and properties. Its structure allows for specific interactions with molecular targets that may not be possible with other similar dipeptides.
Properties
IUPAC Name |
(2S)-2-(3-aminopropanoylamino)-4-methylpentanoic acid |
Source
|
---|---|---|
Source | PubChem | |
URL | https://pubchem.ncbi.nlm.nih.gov | |
Description | Data deposited in or computed by PubChem | |
InChI |
InChI=1S/C9H18N2O3/c1-6(2)5-7(9(13)14)11-8(12)3-4-10/h6-7H,3-5,10H2,1-2H3,(H,11,12)(H,13,14)/t7-/m0/s1 |
Source
|
Source | PubChem | |
URL | https://pubchem.ncbi.nlm.nih.gov | |
Description | Data deposited in or computed by PubChem | |
InChI Key |
YVFFCSSMTOXAQS-ZETCQYMHSA-N |
Source
|
Source | PubChem | |
URL | https://pubchem.ncbi.nlm.nih.gov | |
Description | Data deposited in or computed by PubChem | |
Canonical SMILES |
CC(C)CC(C(=O)O)NC(=O)CCN |
Source
|
Source | PubChem | |
URL | https://pubchem.ncbi.nlm.nih.gov | |
Description | Data deposited in or computed by PubChem | |
Isomeric SMILES |
CC(C)C[C@@H](C(=O)O)NC(=O)CCN |
Source
|
Source | PubChem | |
URL | https://pubchem.ncbi.nlm.nih.gov | |
Description | Data deposited in or computed by PubChem | |
Molecular Formula |
C9H18N2O3 |
Source
|
Source | PubChem | |
URL | https://pubchem.ncbi.nlm.nih.gov | |
Description | Data deposited in or computed by PubChem | |
Molecular Weight |
202.25 g/mol |
Source
|
Source | PubChem | |
URL | https://pubchem.ncbi.nlm.nih.gov | |
Description | Data deposited in or computed by PubChem | |
Q1: What is the impact of orally administered H-Beta-Ala-Leu-OH (Excitin-1) on behavior, and how does it relate to its presence in the brain?
A1: Research indicates that oral administration of this compound in rats leads to increased motor activity, specifically a greater distance traveled and a higher frequency of rearing behaviors in an open field test []. This behavioral change is linked to the compound's ability to cross the blood-brain barrier. Following oral administration, this compound is detectable not only in the plasma but also in various brain regions including the cerebral cortex, hypothalamus, hippocampus, and olfactory bulb []. This suggests that the observed behavioral effects may be attributed to the compound's direct influence on the central nervous system.
Q2: Does the structure of this compound provide clues about its potential mechanism of action in the central nervous system?
A2: While the precise mechanism of action of this compound remains to be fully elucidated, its structure offers some hints. The compound is a dipeptide composed of beta-alanine and L-leucine []. Interestingly, previous research has shown that dipeptides containing beta-alanine and branched-chain amino acids (BCAAs), such as L-leucine, exhibit excitatory effects when administered centrally in chicks []. This suggests that this compound may exert its effects through modulation of neuronal excitability. Furthermore, the study observed alterations in monoamine and amino acid levels in specific brain regions (cerebral cortex and hypothalamus) following this compound administration []. This finding points towards a potential influence on neurotransmitter systems, which warrants further investigation.
Disclaimer and Information on In-Vitro Research Products
Please be aware that all articles and product information presented on BenchChem are intended solely for informational purposes. The products available for purchase on BenchChem are specifically designed for in-vitro studies, which are conducted outside of living organisms. In-vitro studies, derived from the Latin term "in glass," involve experiments performed in controlled laboratory settings using cells or tissues. It is important to note that these products are not categorized as medicines or drugs, and they have not received approval from the FDA for the prevention, treatment, or cure of any medical condition, ailment, or disease. We must emphasize that any form of bodily introduction of these products into humans or animals is strictly prohibited by law. It is essential to adhere to these guidelines to ensure compliance with legal and ethical standards in research and experimentation.