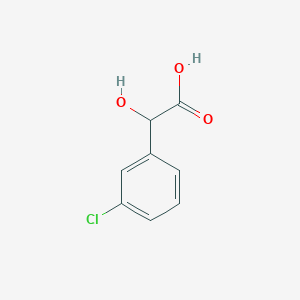
3-Chloromandelic acid
Overview
Description
3-Chloromandelic acid is a chiral compound that belongs to the family of mandelic acids. It is characterized by the presence of a chlorine atom attached to the benzene ring of mandelic acid. This compound is of significant interest due to its applications in various fields, including pharmaceuticals, agrochemicals, and fine chemicals. The presence of the chiral center makes it an important compound for enantioselective synthesis and chiral resolution processes.
Synthetic Routes and Reaction Conditions:
Asymmetric Hydroxylation: One of the methods to synthesize this compound involves the asymmetric hydroxylation of 3-chlorobenzaldehyde. This process typically employs chiral catalysts to achieve high enantioselectivity.
Hydrolysis of 3-Chloromandelonitrile: Another method involves the hydrolysis of 3-chloromandelonitrile using nitrilases.
Industrial Production Methods: The industrial production of this compound often involves the use of biocatalytic processes due to their high specificity and efficiency. For instance, the deracemization of 3-chloromandelonitrile using nitrilases from specific microbial sources has been reported to yield high purity this compound .
Mechanism of Action
Target of Action
It’s structurally similar to mandelic acid and its derivatives, which are known to interact with various enzymes and receptors in the body .
Mode of Action
For instance, mandelic acid derivatives are known to inhibit the release of chemical mediators from sensitized mast cells . This suggests that 3-Chloromandelic acid might also interact with its targets to inhibit certain biochemical reactions, but this needs further investigation.
Biochemical Pathways
Nitrilases, which can hydrolyze nitriles directly to the corresponding carboxylic acids, have been suggested as a potential pathway for the production of mandelic acid and its derivatives . Given the structural similarity, it’s plausible that this compound might be involved in similar pathways.
Result of Action
For instance, mandelic acid derivatives are known to prevent the release of inflammatory chemicals such as histamine from mast cells . This suggests that this compound might have similar anti-inflammatory effects, but this needs further investigation.
Biochemical Analysis
Biochemical Properties
3-Chloromandelic acid plays a crucial role in biochemical reactions, particularly in the synthesis of chiral intermediates. It interacts with several enzymes, including nitrilases and hydroxynitrile lyases. These enzymes facilitate the conversion of this compound into other valuable compounds. For instance, nitrilases catalyze the hydrolysis of nitriles to carboxylic acids, while hydroxynitrile lyases are involved in the formation of cyanohydrins from aldehydes and hydrogen cyanide . The interactions between this compound and these enzymes are essential for its role in various biochemical pathways.
Cellular Effects
This compound influences various cellular processes, including cell signaling pathways, gene expression, and cellular metabolism. It has been observed to affect the activity of certain enzymes and proteins within cells, leading to changes in metabolic flux and gene expression patterns. For example, in recombinant Escherichia coli strains, this compound can be converted to its corresponding amide, which then influences the expression of genes involved in metabolic pathways . These effects highlight the compound’s potential impact on cellular function and its importance in biochemical research.
Molecular Mechanism
The molecular mechanism of this compound involves its interaction with specific biomolecules, leading to enzyme inhibition or activation and changes in gene expression. The compound binds to the active sites of enzymes such as nitrilases and hydroxynitrile lyases, facilitating their catalytic activity. This binding interaction is crucial for the conversion of this compound into other compounds. Additionally, the presence of the chlorine atom in the benzene ring may influence the compound’s binding affinity and specificity towards different enzymes .
Temporal Effects in Laboratory Settings
In laboratory settings, the effects of this compound can change over time due to its stability and degradation. Studies have shown that the compound remains stable under specific conditions, but its activity may decrease over prolonged periods. For instance, in in vitro studies, the catalytic activity of enzymes interacting with this compound may diminish over time, affecting the overall efficiency of biochemical reactions . Understanding these temporal effects is essential for optimizing experimental conditions and ensuring reliable results.
Dosage Effects in Animal Models
The effects of this compound vary with different dosages in animal models. At lower doses, the compound may exhibit beneficial effects, such as enhancing metabolic pathways and improving cellular function. At higher doses, it may cause toxic or adverse effects, including enzyme inhibition and disruption of cellular processes . These dosage-dependent effects are critical for determining the safe and effective use of this compound in biochemical research and potential therapeutic applications.
Transport and Distribution
Within cells and tissues, this compound is transported and distributed through specific transporters and binding proteins. These transporters facilitate the movement of the compound across cellular membranes, ensuring its availability for biochemical reactions. Additionally, binding proteins may influence the localization and accumulation of this compound within specific cellular compartments . Understanding these transport and distribution mechanisms is crucial for optimizing the compound’s use in biochemical research.
Subcellular Localization
The subcellular localization of this compound is influenced by targeting signals and post-translational modifications that direct it to specific compartments or organelles. For instance, the compound may be localized to the cytoplasm or mitochondria, where it participates in metabolic reactions. The specific localization of this compound can affect its activity and function within cells, highlighting the importance of understanding its subcellular distribution .
Chemical Reactions Analysis
3-Chloromandelic acid undergoes various chemical reactions, including:
Oxidation: It can be oxidized to form 3-chlorobenzoylformic acid.
Reduction: Reduction of this compound can yield 3-chlorophenylglycol.
Substitution: The hydroxyl group of this compound can be substituted with other functional groups using appropriate reagents.
Common Reagents and Conditions:
Oxidation: Common oxidizing agents include potassium permanganate and chromium trioxide.
Reduction: Reducing agents such as sodium borohydride or lithium aluminum hydride are used.
Substitution: Reagents like thionyl chloride or phosphorus tribromide can be used for substitution reactions.
Major Products:
Oxidation: 3-Chlorobenzoylformic acid.
Reduction: 3-Chlorophenylglycol.
Substitution: Various substituted derivatives depending on the reagent used.
Scientific Research Applications
3-Chloromandelic acid has several applications in scientific research:
Pharmaceuticals: It is used as an intermediate in the synthesis of various drugs, including cardiovascular agents.
Chiral Resolution: Due to its chiral nature, it is used in the resolution of racemic mixtures in pharmaceutical research.
Agrochemicals: It serves as a building block for the synthesis of agrochemical compounds.
Fine Chemicals: It is used in the synthesis of fine chemicals and specialty chemicals for various industrial applications.
Comparison with Similar Compounds
3-Chloromandelic acid can be compared with other halogenated mandelic acids such as:
- 2-Chloromandelic Acid
- 4-Chloromandelic Acid
- 4-Bromomandelic Acid
- 4-Fluoromandelic Acid
Uniqueness:
- The position of the chlorine atom on the benzene ring (meta position) in this compound imparts unique chemical properties compared to its ortho and para counterparts.
- The specific placement of the chlorine atom affects the compound’s reactivity and its interactions in chiral resolution processes .
Properties
IUPAC Name |
2-(3-chlorophenyl)-2-hydroxyacetic acid | |
---|---|---|
Source | PubChem | |
URL | https://pubchem.ncbi.nlm.nih.gov | |
Description | Data deposited in or computed by PubChem | |
InChI |
InChI=1S/C8H7ClO3/c9-6-3-1-2-5(4-6)7(10)8(11)12/h1-4,7,10H,(H,11,12) | |
Source | PubChem | |
URL | https://pubchem.ncbi.nlm.nih.gov | |
Description | Data deposited in or computed by PubChem | |
InChI Key |
SAMVPMGKGGLIPF-UHFFFAOYSA-N | |
Source | PubChem | |
URL | https://pubchem.ncbi.nlm.nih.gov | |
Description | Data deposited in or computed by PubChem | |
Canonical SMILES |
C1=CC(=CC(=C1)Cl)C(C(=O)O)O | |
Source | PubChem | |
URL | https://pubchem.ncbi.nlm.nih.gov | |
Description | Data deposited in or computed by PubChem | |
Molecular Formula |
C8H7ClO3 | |
Source | PubChem | |
URL | https://pubchem.ncbi.nlm.nih.gov | |
Description | Data deposited in or computed by PubChem | |
DSSTOX Substance ID |
DTXSID801314787 | |
Record name | 3-Chloromandelic acid | |
Source | EPA DSSTox | |
URL | https://comptox.epa.gov/dashboard/DTXSID801314787 | |
Description | DSSTox provides a high quality public chemistry resource for supporting improved predictive toxicology. | |
Molecular Weight |
186.59 g/mol | |
Source | PubChem | |
URL | https://pubchem.ncbi.nlm.nih.gov | |
Description | Data deposited in or computed by PubChem | |
CAS No. |
16273-37-3 | |
Record name | 3-Chloromandelic acid | |
Source | CAS Common Chemistry | |
URL | https://commonchemistry.cas.org/detail?cas_rn=16273-37-3 | |
Description | CAS Common Chemistry is an open community resource for accessing chemical information. Nearly 500,000 chemical substances from CAS REGISTRY cover areas of community interest, including common and frequently regulated chemicals, and those relevant to high school and undergraduate chemistry classes. This chemical information, curated by our expert scientists, is provided in alignment with our mission as a division of the American Chemical Society. | |
Explanation | The data from CAS Common Chemistry is provided under a CC-BY-NC 4.0 license, unless otherwise stated. | |
Record name | 3-Chlorophenylglycolic acid | |
Source | ChemIDplus | |
URL | https://pubchem.ncbi.nlm.nih.gov/substance/?source=chemidplus&sourceid=0016273373 | |
Description | ChemIDplus is a free, web search system that provides access to the structure and nomenclature authority files used for the identification of chemical substances cited in National Library of Medicine (NLM) databases, including the TOXNET system. | |
Record name | 16273-37-3 | |
Source | DTP/NCI | |
URL | https://dtp.cancer.gov/dtpstandard/servlet/dwindex?searchtype=NSC&outputformat=html&searchlist=126599 | |
Description | The NCI Development Therapeutics Program (DTP) provides services and resources to the academic and private-sector research communities worldwide to facilitate the discovery and development of new cancer therapeutic agents. | |
Explanation | Unless otherwise indicated, all text within NCI products is free of copyright and may be reused without our permission. Credit the National Cancer Institute as the source. | |
Record name | 3-Chloromandelic acid | |
Source | EPA DSSTox | |
URL | https://comptox.epa.gov/dashboard/DTXSID801314787 | |
Description | DSSTox provides a high quality public chemistry resource for supporting improved predictive toxicology. | |
Record name | 3-chlorophenylglycolic acid | |
Source | European Chemicals Agency (ECHA) | |
URL | https://echa.europa.eu/substance-information/-/substanceinfo/100.036.690 | |
Description | The European Chemicals Agency (ECHA) is an agency of the European Union which is the driving force among regulatory authorities in implementing the EU's groundbreaking chemicals legislation for the benefit of human health and the environment as well as for innovation and competitiveness. | |
Explanation | Use of the information, documents and data from the ECHA website is subject to the terms and conditions of this Legal Notice, and subject to other binding limitations provided for under applicable law, the information, documents and data made available on the ECHA website may be reproduced, distributed and/or used, totally or in part, for non-commercial purposes provided that ECHA is acknowledged as the source: "Source: European Chemicals Agency, http://echa.europa.eu/". Such acknowledgement must be included in each copy of the material. ECHA permits and encourages organisations and individuals to create links to the ECHA website under the following cumulative conditions: Links can only be made to webpages that provide a link to the Legal Notice page. | |
Synthesis routes and methods
Procedure details
Retrosynthesis Analysis
AI-Powered Synthesis Planning: Our tool employs the Template_relevance Pistachio, Template_relevance Bkms_metabolic, Template_relevance Pistachio_ringbreaker, Template_relevance Reaxys, Template_relevance Reaxys_biocatalysis model, leveraging a vast database of chemical reactions to predict feasible synthetic routes.
One-Step Synthesis Focus: Specifically designed for one-step synthesis, it provides concise and direct routes for your target compounds, streamlining the synthesis process.
Accurate Predictions: Utilizing the extensive PISTACHIO, BKMS_METABOLIC, PISTACHIO_RINGBREAKER, REAXYS, REAXYS_BIOCATALYSIS database, our tool offers high-accuracy predictions, reflecting the latest in chemical research and data.
Strategy Settings
Precursor scoring | Relevance Heuristic |
---|---|
Min. plausibility | 0.01 |
Model | Template_relevance |
Template Set | Pistachio/Bkms_metabolic/Pistachio_ringbreaker/Reaxys/Reaxys_biocatalysis |
Top-N result to add to graph | 6 |
Feasible Synthetic Routes
Q1: What polymorphic forms of 3-Chloromandelic acid exist?
A1: this compound exhibits polymorphism, meaning it can exist in multiple crystalline forms. Research has identified polymorphs for both the enantiomer and the racemate of 3-ClMA. [, , ] These polymorphic forms can influence physicochemical properties relevant for separation and crystallization processes.
Q2: How does the solubility of this compound vary with temperature and solvent?
A2: The solubility of 3-ClMA is highly dependent on temperature, particularly for the pure enantiomers. [] Studies demonstrate significant increases in solubility with rising temperatures in solvents like water. [] Furthermore, the choice of solvent considerably impacts solubility. For example, 3-ClMA exhibits different solubility profiles in water compared to toluene. [, ]
Q3: Can you elaborate on the ternary phase behavior of this compound?
A3: Ternary phase diagrams of 3-ClMA, incorporating a solvent in addition to the enantiomers, reveal valuable information about solubility and potential separation strategies. [, , , ] Notably, the eutectic composition, a crucial parameter for enantiomeric separation, demonstrates temperature dependence in the 3-ClMA system. [] This characteristic shift in eutectic composition within the ternary phase diagram presents opportunities for enantiomeric purification. []
Q4: How accurately can thermodynamic models predict the solubility of this compound?
A4: Thermodynamic models, such as UNIQUAC and NRTL, have been successfully employed to predict the solubility of 3-ClMA with good agreement to experimental data. [, ] These models are valuable tools for predicting solubility across different temperatures and solvent compositions, which can guide experimental design and optimize separation processes.
Q5: Have there been attempts to resolve the enantiomers of this compound?
A5: Yes, several studies have explored the enantioseparation of 3-ClMA. Researchers have successfully resolved 3-ClMA using resolving agents like threo-(1S,2S)-2-amino-l-p-nitrophenyl-1,3-propanediol [(S,S)-SA] and levetiracetam (LEV). [, ] These studies highlight the impact of factors such as solvent type, molar ratios, and temperature on resolution efficiency.
Q6: What is the role of intermolecular interactions in the crystallization of this compound?
A6: Intermolecular interactions play a critical role in the crystallization behavior of 3-ClMA. Studies analyzing crystal structures point to the significance of hydrogen bonding, halogen bonding, and van der Waals forces in dictating crystal packing. [, ] Understanding these interactions is crucial for designing effective chiral separation processes based on crystallization.
Q7: How does the substitution pattern on mandelic acid derivatives, like this compound, influence crystallization?
A7: The type and position of substituents on mandelic acid derivatives significantly influence crystallization outcomes. Research indicates that substituents can affect not only the crystal configuration but also the efficiency of co-crystal resolution. [] This observation highlights the importance of understanding structure-property relationships in chiral molecules for designing effective separation strategies.
Q8: Have there been any studies on the supercritical fluid chromatography of this compound?
A8: Yes, supercritical fluid chromatography (SFC) has been employed for the enantiomeric separation of 3-ClMA and other mandelic acid derivatives. [] The research investigated the influence of various parameters on separation efficiency, including modifier type, temperature, and pressure. SFC offers advantages over traditional HPLC methods in terms of speed and efficiency for enantiomeric separation. []
Q9: What insights do computational studies provide into the crystallization behavior of this compound?
A9: Computational studies, such as crystal structure prediction (CSP) and molecular dynamics simulations, have provided valuable insights into the crystallization of 3-ClMA. [, ] These studies highlight the complexity of the crystal energy landscape and suggest that kinetic factors, rather than solely thermodynamic considerations, play a significant role in determining which crystal forms are obtained experimentally. [, ]
Disclaimer and Information on In-Vitro Research Products
Please be aware that all articles and product information presented on BenchChem are intended solely for informational purposes. The products available for purchase on BenchChem are specifically designed for in-vitro studies, which are conducted outside of living organisms. In-vitro studies, derived from the Latin term "in glass," involve experiments performed in controlled laboratory settings using cells or tissues. It is important to note that these products are not categorized as medicines or drugs, and they have not received approval from the FDA for the prevention, treatment, or cure of any medical condition, ailment, or disease. We must emphasize that any form of bodily introduction of these products into humans or animals is strictly prohibited by law. It is essential to adhere to these guidelines to ensure compliance with legal and ethical standards in research and experimentation.