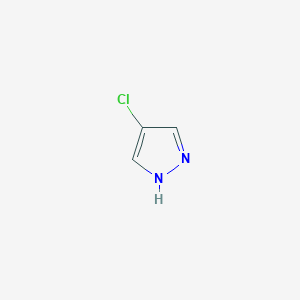
4-Chloro-1H-pyrazole
Overview
Description
4-Chloro-1H-pyrazole is a heterocyclic compound characterized by a five-membered ring structure containing two nitrogen atoms and one chlorine atom attached to the fourth carbon. This compound is part of the pyrazole family, which is known for its versatility in organic synthesis and medicinal chemistry
Mechanism of Action
Target of Action
4-Chloropyrazole, also known as 4-Chloro-1H-pyrazole, is a member of the pyrazole family, a class of N-heterocyclic compounds Pyrazole derivatives are known for their diverse pharmacological effects, including potent antileishmanial and antimalarial activities .
Mode of Action
Pyrazoles can act as weak bases or acids, with possible strength highly dependent on the nature of their substituent groups . The introduction of halopyrazole groups can significantly improve the insecticidal activity of matrine .
Biochemical Pathways
Pyrazole-containing compounds are influential due to their applicability and versatility as synthetic intermediates in preparing relevant chemicals in biological, physical-chemical, material science, and industrial fields .
Pharmacokinetics
The optimization of the adme properties of a drug molecule is often the most difficult and challenging part of the whole drug discovery process .
Result of Action
Pyrazole derivatives are known for their diverse pharmacological effects .
Action Environment
Safety data sheets recommend handling the compound with care to prevent it from being discharged into the environment without proper treatment .
Biochemical Analysis
Biochemical Properties
4-Chloropyrazole plays a significant role in biochemical reactions. It interacts with various enzymes, proteins, and other biomolecules
Cellular Effects
The effects of 4-Chloropyrazole on various types of cells and cellular processes are complex and multifaceted. It influences cell function, including impacts on cell signaling pathways, gene expression, and cellular metabolism
Molecular Mechanism
The molecular mechanism of action of 4-Chloropyrazole involves a range of interactions at the molecular level. These include binding interactions with biomolecules, potential enzyme inhibition or activation, and changes in gene expression . The exact mechanisms are still being explored in scientific research.
Temporal Effects in Laboratory Settings
In laboratory settings, the effects of 4-Chloropyrazole can change over time. This includes information on the product’s stability, degradation, and any long-term effects on cellular function observed in in vitro or in vivo studies .
Dosage Effects in Animal Models
The effects of 4-Chloropyrazole can vary with different dosages in animal models . This includes any threshold effects observed in these studies, as well as any toxic or adverse effects at high doses.
Metabolic Pathways
4-Chloropyrazole is involved in various metabolic pathways. It interacts with several enzymes and cofactors, and can also influence metabolic flux or metabolite levels . The specifics of these pathways and interactions are currently under study.
Transport and Distribution
4-Chloropyrazole is transported and distributed within cells and tissues in a manner that is still being researched. It may interact with various transporters or binding proteins, and these interactions could influence its localization or accumulation .
Subcellular Localization
The subcellular localization of 4-Chloropyrazole and any effects on its activity or function are areas of active research. This could include any targeting signals or post-translational modifications that direct it to specific compartments or organelles .
Preparation Methods
Synthetic Routes and Reaction Conditions: 4-Chloro-1H-pyrazole can be synthesized through several methods. One common approach involves the cyclocondensation of hydrazine with 1,3-dicarbonyl compounds, followed by chlorination. Another method includes the reaction of 4-chloropyrazole with appropriate reagents under controlled conditions .
Industrial Production Methods: In industrial settings, the synthesis of this compound often involves the use of advanced techniques such as microwave-assisted synthesis and green chemistry approaches to enhance yield and reduce environmental impact . The use of catalysts and optimized reaction conditions is crucial for large-scale production.
Chemical Reactions Analysis
Types of Reactions: 4-Chloro-1H-pyrazole undergoes various chemical reactions, including:
Substitution Reactions: The chlorine atom can be substituted with other functional groups using nucleophilic reagents.
Oxidation and Reduction: The compound can be oxidized or reduced under specific conditions to form different derivatives.
Cyclization Reactions: It can participate in cyclization reactions to form more complex heterocyclic structures.
Common Reagents and Conditions:
Nucleophilic Substitution: Reagents such as sodium hydroxide or potassium carbonate are commonly used.
Oxidation: Oxidizing agents like hydrogen peroxide or potassium permanganate.
Reduction: Reducing agents such as sodium borohydride or lithium aluminum hydride.
Major Products: The major products formed from these reactions include various substituted pyrazoles, which have significant applications in medicinal chemistry and material science .
Scientific Research Applications
4-Chloro-1H-pyrazole has a wide range of applications in scientific research:
Chemistry: It serves as a building block for the synthesis of more complex heterocyclic compounds.
Biology: It is used in the development of bioactive molecules with potential therapeutic effects.
Medicine: The compound is explored for its potential as an anti-inflammatory, antimicrobial, and anticancer agent.
Industry: It is used in the production of agrochemicals, dyes, and polymers
Comparison with Similar Compounds
4-Bromo-1H-pyrazole: Similar structure but with a bromine atom instead of chlorine.
4-Iodo-1H-pyrazole: Contains an iodine atom, leading to different reactivity.
3,5-Dichloro-1H-pyrazole: Contains two chlorine atoms, affecting its chemical properties
Uniqueness: Its versatility in undergoing various chemical reactions and its wide range of applications in different fields make it a valuable compound in scientific research .
Properties
IUPAC Name |
4-chloro-1H-pyrazole | |
---|---|---|
Source | PubChem | |
URL | https://pubchem.ncbi.nlm.nih.gov | |
Description | Data deposited in or computed by PubChem | |
InChI |
InChI=1S/C3H3ClN2/c4-3-1-5-6-2-3/h1-2H,(H,5,6) | |
Source | PubChem | |
URL | https://pubchem.ncbi.nlm.nih.gov | |
Description | Data deposited in or computed by PubChem | |
InChI Key |
BADSZRMNXWLUKO-UHFFFAOYSA-N | |
Source | PubChem | |
URL | https://pubchem.ncbi.nlm.nih.gov | |
Description | Data deposited in or computed by PubChem | |
Canonical SMILES |
C1=C(C=NN1)Cl | |
Source | PubChem | |
URL | https://pubchem.ncbi.nlm.nih.gov | |
Description | Data deposited in or computed by PubChem | |
Molecular Formula |
C3H3ClN2 | |
Source | PubChem | |
URL | https://pubchem.ncbi.nlm.nih.gov | |
Description | Data deposited in or computed by PubChem | |
DSSTOX Substance ID |
DTXSID90166523 | |
Record name | 1H-Pyrazole, 4-chloro- (9CI) | |
Source | EPA DSSTox | |
URL | https://comptox.epa.gov/dashboard/DTXSID90166523 | |
Description | DSSTox provides a high quality public chemistry resource for supporting improved predictive toxicology. | |
Molecular Weight |
102.52 g/mol | |
Source | PubChem | |
URL | https://pubchem.ncbi.nlm.nih.gov | |
Description | Data deposited in or computed by PubChem | |
CAS No. |
15878-00-9 | |
Record name | 4-Chloropyrazole | |
Source | CAS Common Chemistry | |
URL | https://commonchemistry.cas.org/detail?cas_rn=15878-00-9 | |
Description | CAS Common Chemistry is an open community resource for accessing chemical information. Nearly 500,000 chemical substances from CAS REGISTRY cover areas of community interest, including common and frequently regulated chemicals, and those relevant to high school and undergraduate chemistry classes. This chemical information, curated by our expert scientists, is provided in alignment with our mission as a division of the American Chemical Society. | |
Explanation | The data from CAS Common Chemistry is provided under a CC-BY-NC 4.0 license, unless otherwise stated. | |
Record name | Pyrazole, 4-chloro- | |
Source | ChemIDplus | |
URL | https://pubchem.ncbi.nlm.nih.gov/substance/?source=chemidplus&sourceid=0015878009 | |
Description | ChemIDplus is a free, web search system that provides access to the structure and nomenclature authority files used for the identification of chemical substances cited in National Library of Medicine (NLM) databases, including the TOXNET system. | |
Record name | 1H-Pyrazole, 4-chloro- (9CI) | |
Source | EPA DSSTox | |
URL | https://comptox.epa.gov/dashboard/DTXSID90166523 | |
Description | DSSTox provides a high quality public chemistry resource for supporting improved predictive toxicology. | |
Record name | 4-Chloropyrazole | |
Source | European Chemicals Agency (ECHA) | |
URL | https://echa.europa.eu/information-on-chemicals | |
Description | The European Chemicals Agency (ECHA) is an agency of the European Union which is the driving force among regulatory authorities in implementing the EU's groundbreaking chemicals legislation for the benefit of human health and the environment as well as for innovation and competitiveness. | |
Explanation | Use of the information, documents and data from the ECHA website is subject to the terms and conditions of this Legal Notice, and subject to other binding limitations provided for under applicable law, the information, documents and data made available on the ECHA website may be reproduced, distributed and/or used, totally or in part, for non-commercial purposes provided that ECHA is acknowledged as the source: "Source: European Chemicals Agency, http://echa.europa.eu/". Such acknowledgement must be included in each copy of the material. ECHA permits and encourages organisations and individuals to create links to the ECHA website under the following cumulative conditions: Links can only be made to webpages that provide a link to the Legal Notice page. | |
Retrosynthesis Analysis
AI-Powered Synthesis Planning: Our tool employs the Template_relevance Pistachio, Template_relevance Bkms_metabolic, Template_relevance Pistachio_ringbreaker, Template_relevance Reaxys, Template_relevance Reaxys_biocatalysis model, leveraging a vast database of chemical reactions to predict feasible synthetic routes.
One-Step Synthesis Focus: Specifically designed for one-step synthesis, it provides concise and direct routes for your target compounds, streamlining the synthesis process.
Accurate Predictions: Utilizing the extensive PISTACHIO, BKMS_METABOLIC, PISTACHIO_RINGBREAKER, REAXYS, REAXYS_BIOCATALYSIS database, our tool offers high-accuracy predictions, reflecting the latest in chemical research and data.
Strategy Settings
Precursor scoring | Relevance Heuristic |
---|---|
Min. plausibility | 0.01 |
Model | Template_relevance |
Template Set | Pistachio/Bkms_metabolic/Pistachio_ringbreaker/Reaxys/Reaxys_biocatalysis |
Top-N result to add to graph | 6 |
Feasible Synthetic Routes
Q1: What is the molecular formula, weight, and spectroscopic data for 4-Chloropyrazole?
A1: 4-Chloropyrazole has the molecular formula C3H3ClN2 and a molecular weight of 102.53 g/mol. Spectroscopic characterization data includes:
- IR: Characteristic peaks have been reported for N–H stretching and ring vibrations. [, ]
- 1H NMR: Provides distinct signals for the pyrazole ring protons, influenced by the chlorine substituent. [, ]
- 13C NMR: Shows characteristic peaks for the carbon atoms in the pyrazole ring. []
Q2: Does 4-Chloropyrazole exhibit any catalytic properties?
A3: While 4-Chloropyrazole itself has not been widely explored for its catalytic activity, its derivatives, particularly metal complexes, have shown potential. For instance, ruthenium complexes with 4-chloropyrazole ligands demonstrate light-activated cytotoxicity against cancer cells. [] This activity highlights the potential of 4-chloropyrazole-based metal complexes in photoactivated chemotherapy.
Q3: Has computational chemistry been employed to study 4-Chloropyrazole?
A4: Yes, Density Functional Theory (DFT) calculations have been used to analyze the optimized structure of 4-Chloropyrazole. [] This study provided insights into the molecule's electronic structure, including the highest occupied molecular orbital (HOMO), lowest unoccupied molecular orbital (LUMO), molecular electrostatic potential, and natural bond orbitals. These findings contribute to a deeper understanding of the molecule's reactivity and potential applications.
Q4: How do modifications to the 4-Chloropyrazole structure influence its activity?
A5: Research on 4-chloropyrazole-based pyridine derivatives as fungicide candidates reveals that structural modifications significantly impact their antifungal activity. [] The position and nature of substituents on the pyridine ring influenced the compounds' ability to inhibit fungal sterol biosynthesis, indicating a strong structure-activity relationship.
Q5: Are there strategies to enhance the stability or bioavailability of 4-Chloropyrazole?
A6: While specific formulation strategies for 4-Chloropyrazole are not extensively documented in the provided research, its use in synthesizing various derivatives suggests potential modifications. For example, the transformation of 4-chloropyrazole into 1-methyl-3,5-dinitropyrazole-4-nitrate, an energetic material with enhanced properties, highlights the potential for derivatization to achieve desired stability and performance characteristics. []
Q6: What are some common synthetic routes to obtain 4-Chloropyrazole?
A6: Several methods for synthesizing 4-chloropyrazole have been reported:
- Chlorination of Pyrazole: This approach uses trichloroisocyanuric acid as a chlorinating agent under solvent-free mechanochemical conditions. []
- From Propargyl Amine: A multi-step synthesis involves the conversion of propargyl amine to pyrazole N-oxide, followed by chlorination using DMF and POCl3. [, ]
- From α-Chloro-β-ketodimethoxyacetals: This method allows for the preparation of 4-chloropyrazoles from readily available starting materials. [, ]
Q7: What types of reactions is 4-Chloropyrazole known to undergo?
A7: 4-Chloropyrazole is a versatile building block for various transformations:
- Nucleophilic Substitution: The chlorine atom can be displaced by nucleophiles, exemplified by the synthesis of 1-methyl-3,5-dinitropyrazole-4-nitrate. []
- Palladium-Catalyzed Arylation: This reaction enables the regioselective introduction of aryl groups at the 5-position of the pyrazole ring. [, ]
- Nitration: 4-Chloropyrazole serves as a precursor for synthesizing energetic materials like 4-chloro-3,5-dinitropyrazole via nitration reactions. []
Q8: What are some of the key applications of 4-Chloropyrazole and its derivatives?
A8: 4-Chloropyrazole and its derivatives exhibit promise in various fields:
- Energetic Materials: It acts as a precursor to energetic compounds like 4-chloro-3,5-dinitropyrazole and 1-methyl-3,5-dinitropyrazole-4-nitrate. [, ]
- Fungicides: 4-Chloropyrazole-based pyridine derivatives display potent antifungal activities. []
- Pharmaceuticals: The core structure of 4-chloropyrazole is found in various pharmaceuticals and bioactive compounds. [, ]
- Photoactivated Chemotherapy: Ruthenium complexes with 4-chloropyrazole ligands show potential as photoactivated anticancer agents. []
Disclaimer and Information on In-Vitro Research Products
Please be aware that all articles and product information presented on BenchChem are intended solely for informational purposes. The products available for purchase on BenchChem are specifically designed for in-vitro studies, which are conducted outside of living organisms. In-vitro studies, derived from the Latin term "in glass," involve experiments performed in controlled laboratory settings using cells or tissues. It is important to note that these products are not categorized as medicines or drugs, and they have not received approval from the FDA for the prevention, treatment, or cure of any medical condition, ailment, or disease. We must emphasize that any form of bodily introduction of these products into humans or animals is strictly prohibited by law. It is essential to adhere to these guidelines to ensure compliance with legal and ethical standards in research and experimentation.