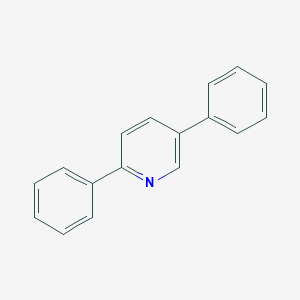
2,5-Diphenylpyridine
Overview
Description
2,5-Diphenylpyridine is an organic compound with the molecular formula C₁₇H₁₃N. It is a derivative of pyridine, where two phenyl groups are substituted at the 2 and 5 positions of the pyridine ring.
Mechanism of Action
Target of Action
2,5-Diphenylpyridine is a ligand that can act as a chelate ligand . It has been used as a model system for the transport properties of hydrogen bonds in group P2 . .
Mode of Action
It has been shown to have fluorescent properties . This suggests that it may interact with its targets in a way that alters their fluorescence, potentially making it useful as a probe in biochemical studies .
Biochemical Pathways
For example, pyridine derivatives occur ubiquitously as pyridoxine (vitamin B6) and vitamin B3 (primarily in the form of niacin [nicotinic acid or pyridine-3-carboxylic acid] and nicotinamide [3-pyridinecarboxamide]) which is used in the synthesis of nicotinamide adenine dinucleotide (NAD+) and nicotinamide adenine dinucleotide phosphate (NADP+) .
Pharmacokinetics
It is known that the compound is a solid at room temperature , which could impact its bioavailability.
Result of Action
It has been shown to have fluorescent properties , suggesting that it may be used as a photophysical probe to study biochemical properties such as ester linkages .
Preparation Methods
Synthetic Routes and Reaction Conditions: 2,5-Diphenylpyridine can be synthesized through several methods. One common approach involves the Suzuki-Miyaura cross-coupling reaction. This method uses a palladium catalyst to couple a halogenated pyridine with a phenylboronic acid . The reaction typically occurs in the presence of a base, such as potassium carbonate, and a solvent like toluene or ethanol. The reaction conditions are generally mild, with temperatures ranging from 80°C to 100°C.
Industrial Production Methods: In an industrial setting, the synthesis of this compound may involve large-scale batch reactors where the reagents are combined under controlled conditions. The use of continuous flow reactors can also enhance the efficiency and yield of the production process. The final product is usually purified through recrystallization or chromatography techniques to achieve high purity levels .
Chemical Reactions Analysis
Types of Reactions: 2,5-Diphenylpyridine undergoes various chemical reactions, including:
Oxidation: This compound can be oxidized using strong oxidizing agents like potassium permanganate or chromium trioxide, leading to the formation of corresponding pyridine N-oxides.
Reduction: Reduction reactions can be carried out using reducing agents such as lithium aluminum hydride or sodium borohydride, which can reduce the pyridine ring to a piperidine derivative.
Common Reagents and Conditions:
Oxidation: Potassium permanganate in acidic medium.
Reduction: Lithium aluminum hydride in anhydrous ether.
Substitution: Nitration using nitric acid and sulfuric acid mixture.
Major Products Formed:
Oxidation: Pyridine N-oxides.
Reduction: Piperidine derivatives.
Substitution: Nitro, halogen, or alkyl-substituted diphenylpyridines.
Scientific Research Applications
2,5-Diphenylpyridine has a wide range of applications in scientific research:
Comparison with Similar Compounds
- 2,4-Diphenylpyridine
- 2,6-Diphenylpyridine
- 3,5-Diphenylpyridine
Comparison: 2,5-Diphenylpyridine is unique due to the specific positioning of the phenyl groups, which influences its electronic and steric properties. Compared to 2,4-Diphenylpyridine and 2,6-Diphenylpyridine, the 2,5-isomer exhibits different reactivity and binding characteristics in coordination chemistry. The 3,5-Diphenylpyridine, on the other hand, has a different substitution pattern, leading to variations in its chemical behavior and applications .
Properties
IUPAC Name |
2,5-diphenylpyridine | |
---|---|---|
Source | PubChem | |
URL | https://pubchem.ncbi.nlm.nih.gov | |
Description | Data deposited in or computed by PubChem | |
InChI |
InChI=1S/C17H13N/c1-3-7-14(8-4-1)16-11-12-17(18-13-16)15-9-5-2-6-10-15/h1-13H | |
Source | PubChem | |
URL | https://pubchem.ncbi.nlm.nih.gov | |
Description | Data deposited in or computed by PubChem | |
InChI Key |
MOSQXYPUMBJMRR-UHFFFAOYSA-N | |
Source | PubChem | |
URL | https://pubchem.ncbi.nlm.nih.gov | |
Description | Data deposited in or computed by PubChem | |
Canonical SMILES |
C1=CC=C(C=C1)C2=CN=C(C=C2)C3=CC=CC=C3 | |
Source | PubChem | |
URL | https://pubchem.ncbi.nlm.nih.gov | |
Description | Data deposited in or computed by PubChem | |
Molecular Formula |
C17H13N | |
Source | PubChem | |
URL | https://pubchem.ncbi.nlm.nih.gov | |
Description | Data deposited in or computed by PubChem | |
DSSTOX Substance ID |
DTXSID60376516 | |
Record name | 2,5-diphenylpyridine | |
Source | EPA DSSTox | |
URL | https://comptox.epa.gov/dashboard/DTXSID60376516 | |
Description | DSSTox provides a high quality public chemistry resource for supporting improved predictive toxicology. | |
Molecular Weight |
231.29 g/mol | |
Source | PubChem | |
URL | https://pubchem.ncbi.nlm.nih.gov | |
Description | Data deposited in or computed by PubChem | |
CAS No. |
15827-72-2 | |
Record name | 2,5-diphenylpyridine | |
Source | EPA DSSTox | |
URL | https://comptox.epa.gov/dashboard/DTXSID60376516 | |
Description | DSSTox provides a high quality public chemistry resource for supporting improved predictive toxicology. | |
Synthesis routes and methods
Procedure details
Retrosynthesis Analysis
AI-Powered Synthesis Planning: Our tool employs the Template_relevance Pistachio, Template_relevance Bkms_metabolic, Template_relevance Pistachio_ringbreaker, Template_relevance Reaxys, Template_relevance Reaxys_biocatalysis model, leveraging a vast database of chemical reactions to predict feasible synthetic routes.
One-Step Synthesis Focus: Specifically designed for one-step synthesis, it provides concise and direct routes for your target compounds, streamlining the synthesis process.
Accurate Predictions: Utilizing the extensive PISTACHIO, BKMS_METABOLIC, PISTACHIO_RINGBREAKER, REAXYS, REAXYS_BIOCATALYSIS database, our tool offers high-accuracy predictions, reflecting the latest in chemical research and data.
Strategy Settings
Precursor scoring | Relevance Heuristic |
---|---|
Min. plausibility | 0.01 |
Model | Template_relevance |
Template Set | Pistachio/Bkms_metabolic/Pistachio_ringbreaker/Reaxys/Reaxys_biocatalysis |
Top-N result to add to graph | 6 |
Feasible Synthetic Routes
Q1: What are the structural characteristics of 2,5-diphenylpyridine?
A1: this compound is an organic compound with the molecular formula C17H13N and a molecular weight of 231.29 g/mol []. It consists of a central pyridine ring (a six-membered aromatic ring with one nitrogen atom) with phenyl groups (C6H5) attached at the 2 and 5 positions. Spectroscopic data, including UV-Vis absorption and fluorescence emission spectra, are often used to characterize these compounds [, ].
Q2: How does this compound interact with metals to form complexes relevant to OLED technology?
A2: this compound can act as a ligand, coordinating to transition metals like iridium(III) and platinum(II) [, ]. These metals have high spin-orbit coupling constants, which facilitate phosphorescence – the emission of light from a triplet excited state []. In the context of OLEDs, this phosphorescence is crucial for achieving high device efficiencies [].
Q3: What are the advantages of incorporating this compound derivatives into iridium(III) complexes for OLED applications?
A3: Studies have demonstrated that iridium(III) complexes containing modified this compound ligands exhibit promising properties for OLEDs. For instance, introducing alkoxy chains to the phenyl rings can induce liquid-crystalline behavior, potentially enhancing charge transport within the device []. Additionally, specific modifications can tune the emission color [] and improve the complexes' thermal stability, a crucial factor for device longevity [].
Q4: Are there any limitations associated with using this compound-based complexes in OLEDs?
A4: While promising, this compound-based complexes face challenges. For example, while platinum(II) complexes often exhibit room-temperature phosphorescence, their palladium(II) counterparts, despite structural similarities, do not always show this desirable property []. This highlights the importance of careful metal selection. Furthermore, achieving the desired morphology and charge transport properties in the active layer of OLEDs can be challenging and requires precise control over the self-assembly of these complexes [].
Q5: How is computational chemistry used to study this compound and its derivatives?
A5: Computational chemistry plays a vital role in understanding the properties of this compound derivatives. Density Functional Theory (DFT) calculations help optimize molecular geometries, predict vibrational frequencies, and analyze electronic structures []. These insights are crucial for understanding factors like emission color, redox properties, and the stability of metal complexes formed with these ligands.
Q6: What is the significance of Structure-Activity Relationships (SAR) in this compound research?
A6: SAR studies are essential for optimizing the performance of this compound derivatives in various applications. For example, researchers have explored how modifications to the phenyl rings, such as introducing different substituents or varying the length and number of alkoxy chains, impact the liquid-crystalline behavior, emission color, and charge transport properties of the resulting complexes [, , ]. This systematic approach enables the fine-tuning of material properties for specific applications.
Q7: Beyond OLEDs, are there other applications of this compound derivatives?
A7: While OLEDs represent a significant area of interest, this compound derivatives have potential in other fields. Their fluorescent properties make them suitable for applications in bioimaging and sensing []. Moreover, the ability of these compounds to coordinate with metals opens possibilities for catalytic applications, although further research is needed to explore this avenue.
Disclaimer and Information on In-Vitro Research Products
Please be aware that all articles and product information presented on BenchChem are intended solely for informational purposes. The products available for purchase on BenchChem are specifically designed for in-vitro studies, which are conducted outside of living organisms. In-vitro studies, derived from the Latin term "in glass," involve experiments performed in controlled laboratory settings using cells or tissues. It is important to note that these products are not categorized as medicines or drugs, and they have not received approval from the FDA for the prevention, treatment, or cure of any medical condition, ailment, or disease. We must emphasize that any form of bodily introduction of these products into humans or animals is strictly prohibited by law. It is essential to adhere to these guidelines to ensure compliance with legal and ethical standards in research and experimentation.