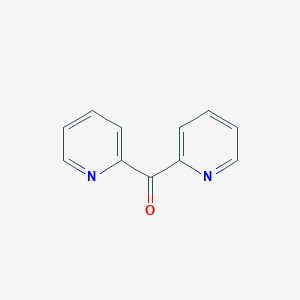
di-2-Pyridyl ketone
Overview
Description
Mechanism of Action
Target of Action
Di-2-Pyridyl ketone is a versatile polydentate ligand that has been extensively studied for its reactions with transition metal and lanthanide ions . The primary targets of this compound are cancer cells, specifically SK-N-MC neuroepithelioma cells .
Mode of Action
The Schiff base ligands derived from this compound exhibit antiproliferative activity in SK-N-MC neuroepithelioma (cancer) cells . This compound interacts with its targets by promoting the production of reactive oxygen species (ROS), leading to caspase-dependent apoptotic cell death .
Biochemical Pathways
The this compound is involved in the synthesis of unusual {Ni(II)(3)Ln(III)(μ-OR)(6)}(3+) complexes with a “star” topology . Its monoanionic form has been used in the synthesis of triangular Ni(2)M (M = lanthanide, Y) complexes . These complexes have been found to exhibit anticancer activity .
Pharmacokinetics
It is known that the compound has low solubility in water , which could impact its bioavailability.
Result of Action
The result of this compound’s action is the induction of apoptosis in cancer cells . This is achieved through the promotion of reactive oxygen species (ROS) production, leading to caspase-dependent apoptotic cell death .
Action Environment
Environmental factors can influence the action, efficacy, and stability of this compound. For instance, the compound’s solubility in water can affect its bioavailability and thus its efficacy . Additionally, the compound’s interaction with other elements, such as copper and lanthanide ions, can also influence its action .
Biochemical Analysis
Biochemical Properties
Di-2-Pyridyl Ketone interacts with various enzymes, proteins, and other biomolecules. The Schiff base ligands derived from this compound exhibit antiproliferative activity in SK-N-MC neuroepithelioma (cancer) cells .
Cellular Effects
It is known that the compound influences cell function, including impacts on cell signaling pathways, gene expression, and cellular metabolism .
Molecular Mechanism
At the molecular level, this compound exerts its effects through binding interactions with biomolecules, enzyme inhibition or activation, and changes in gene expression .
Temporal Effects in Laboratory Settings
In laboratory settings, the effects of this compound change over time. Information on the product’s stability, degradation, and any long-term effects on cellular function observed in in vitro or in vivo studies is currently being researched .
Dosage Effects in Animal Models
The effects of this compound vary with different dosages in animal models. Studies are ongoing to determine any threshold effects observed in these studies, as well as any toxic or adverse effects at high doses .
Metabolic Pathways
This compound is involved in various metabolic pathways. It interacts with several enzymes or cofactors, and it may also affect metabolic flux or metabolite levels .
Subcellular Localization
The subcellular localization of this compound and its effects on activity or function are areas of active research. This includes any targeting signals or post-translational modifications that direct it to specific compartments or organelles .
Preparation Methods
Synthetic Routes and Reaction Conditions
Di-2-Pyridyl ketone can be synthesized through several methods. One common synthetic route involves the reaction of 2-bromopyridine with n-butyllithium in tetrahydrofuran at -78°C, followed by the addition of ethyl chloroformate . Another method involves the use of 2-pyridinecarboxaldehyde as a starting material .
Industrial Production Methods
. The production typically involves standard organic synthesis techniques and purification processes to achieve high purity levels.
Chemical Reactions Analysis
Types of Reactions
Di-2-Pyridyl ketone undergoes various chemical reactions, including:
Oxidation: The compound can be oxidized to form corresponding oximes.
Reduction: Under reducing conditions, the carbonyl group can be converted to a hydroxyl group.
Substitution: It can react with halides and other nucleophiles to form substituted products.
Common Reagents and Conditions
Oxidation: Common oxidizing agents include hydrogen peroxide and peracids.
Reduction: Reducing agents such as sodium borohydride or lithium aluminum hydride are typically used.
Substitution: Reactions with halides often require the presence of a base such as sodium hydroxide.
Major Products Formed
Oximes: Formed through the reaction with hydroxylamine.
Hydroxyl Derivatives: Formed under reducing conditions.
Substituted Pyridyl Ketones: Formed through nucleophilic substitution reactions.
Scientific Research Applications
Di-2-Pyridyl ketone has a wide range of applications in scientific research:
Comparison with Similar Compounds
Di-2-Pyridyl ketone can be compared with other similar compounds such as:
2-Acetylpyridine: Similar in structure but with different coordination properties.
2-Benzoylpyridine: Another related compound with distinct chemical behavior.
This compound oxime: A derivative with an additional oxime group, used in solvent extraction of metal ions.
This compound is unique due to its versatile coordination modes and the ability to form stable complexes with a wide range of metal ions, making it a valuable ligand in various fields of research .
Properties
IUPAC Name |
dipyridin-2-ylmethanone | |
---|---|---|
Source | PubChem | |
URL | https://pubchem.ncbi.nlm.nih.gov | |
Description | Data deposited in or computed by PubChem | |
InChI |
InChI=1S/C11H8N2O/c14-11(9-5-1-3-7-12-9)10-6-2-4-8-13-10/h1-8H | |
Source | PubChem | |
URL | https://pubchem.ncbi.nlm.nih.gov | |
Description | Data deposited in or computed by PubChem | |
InChI Key |
QPOWUYJWCJRLEE-UHFFFAOYSA-N | |
Source | PubChem | |
URL | https://pubchem.ncbi.nlm.nih.gov | |
Description | Data deposited in or computed by PubChem | |
Canonical SMILES |
C1=CC=NC(=C1)C(=O)C2=CC=CC=N2 | |
Source | PubChem | |
URL | https://pubchem.ncbi.nlm.nih.gov | |
Description | Data deposited in or computed by PubChem | |
Molecular Formula |
C11H8N2O | |
Source | PubChem | |
URL | https://pubchem.ncbi.nlm.nih.gov | |
Description | Data deposited in or computed by PubChem | |
DSSTOX Substance ID |
DTXSID20173068 | |
Record name | Bis(2-pyridyl) ketone | |
Source | EPA DSSTox | |
URL | https://comptox.epa.gov/dashboard/DTXSID20173068 | |
Description | DSSTox provides a high quality public chemistry resource for supporting improved predictive toxicology. | |
Molecular Weight |
184.19 g/mol | |
Source | PubChem | |
URL | https://pubchem.ncbi.nlm.nih.gov | |
Description | Data deposited in or computed by PubChem | |
CAS No. |
19437-26-4 | |
Record name | 2-Pyridyl ketone | |
Source | CAS Common Chemistry | |
URL | https://commonchemistry.cas.org/detail?cas_rn=19437-26-4 | |
Description | CAS Common Chemistry is an open community resource for accessing chemical information. Nearly 500,000 chemical substances from CAS REGISTRY cover areas of community interest, including common and frequently regulated chemicals, and those relevant to high school and undergraduate chemistry classes. This chemical information, curated by our expert scientists, is provided in alignment with our mission as a division of the American Chemical Society. | |
Explanation | The data from CAS Common Chemistry is provided under a CC-BY-NC 4.0 license, unless otherwise stated. | |
Record name | Bis(2-pyridyl) ketone | |
Source | ChemIDplus | |
URL | https://pubchem.ncbi.nlm.nih.gov/substance/?source=chemidplus&sourceid=0019437264 | |
Description | ChemIDplus is a free, web search system that provides access to the structure and nomenclature authority files used for the identification of chemical substances cited in National Library of Medicine (NLM) databases, including the TOXNET system. | |
Record name | 19437-26-4 | |
Source | DTP/NCI | |
URL | https://dtp.cancer.gov/dtpstandard/servlet/dwindex?searchtype=NSC&outputformat=html&searchlist=174057 | |
Description | The NCI Development Therapeutics Program (DTP) provides services and resources to the academic and private-sector research communities worldwide to facilitate the discovery and development of new cancer therapeutic agents. | |
Explanation | Unless otherwise indicated, all text within NCI products is free of copyright and may be reused without our permission. Credit the National Cancer Institute as the source. | |
Record name | Bis(2-pyridyl) ketone | |
Source | EPA DSSTox | |
URL | https://comptox.epa.gov/dashboard/DTXSID20173068 | |
Description | DSSTox provides a high quality public chemistry resource for supporting improved predictive toxicology. | |
Record name | Bis(2-pyridyl) ketone | |
Source | European Chemicals Agency (ECHA) | |
URL | https://echa.europa.eu/substance-information/-/substanceinfo/100.039.137 | |
Description | The European Chemicals Agency (ECHA) is an agency of the European Union which is the driving force among regulatory authorities in implementing the EU's groundbreaking chemicals legislation for the benefit of human health and the environment as well as for innovation and competitiveness. | |
Explanation | Use of the information, documents and data from the ECHA website is subject to the terms and conditions of this Legal Notice, and subject to other binding limitations provided for under applicable law, the information, documents and data made available on the ECHA website may be reproduced, distributed and/or used, totally or in part, for non-commercial purposes provided that ECHA is acknowledged as the source: "Source: European Chemicals Agency, http://echa.europa.eu/". Such acknowledgement must be included in each copy of the material. ECHA permits and encourages organisations and individuals to create links to the ECHA website under the following cumulative conditions: Links can only be made to webpages that provide a link to the Legal Notice page. | |
Retrosynthesis Analysis
AI-Powered Synthesis Planning: Our tool employs the Template_relevance Pistachio, Template_relevance Bkms_metabolic, Template_relevance Pistachio_ringbreaker, Template_relevance Reaxys, Template_relevance Reaxys_biocatalysis model, leveraging a vast database of chemical reactions to predict feasible synthetic routes.
One-Step Synthesis Focus: Specifically designed for one-step synthesis, it provides concise and direct routes for your target compounds, streamlining the synthesis process.
Accurate Predictions: Utilizing the extensive PISTACHIO, BKMS_METABOLIC, PISTACHIO_RINGBREAKER, REAXYS, REAXYS_BIOCATALYSIS database, our tool offers high-accuracy predictions, reflecting the latest in chemical research and data.
Strategy Settings
Precursor scoring | Relevance Heuristic |
---|---|
Min. plausibility | 0.01 |
Model | Template_relevance |
Template Set | Pistachio/Bkms_metabolic/Pistachio_ringbreaker/Reaxys/Reaxys_biocatalysis |
Top-N result to add to graph | 6 |
Feasible Synthetic Routes
Q1: What is the molecular formula, weight, and key spectroscopic data for di-2-pyridyl ketone?
A1: this compound has the molecular formula C11H8N2O and a molecular weight of 184.19 g/mol. Spectroscopically, it is characterized by a strong infrared absorption band for the carbonyl group (C=O) around 1670 cm-1. In 1H NMR spectroscopy, the aromatic protons of the pyridyl rings typically appear in the range of 7-9 ppm. []
Q2: How does the presence of the carbonyl group in DPK influence its reactivity?
A2: The carbonyl group, being polarized with an electrophilic carbon atom, makes DPK susceptible to nucleophilic attack. This property enables DPK to undergo a range of reactions, including the formation of gem-diols, hemiacetals, and hydrazones. []
Q3: What is unique about the coordination behavior of this compound?
A3: DPK exhibits diverse coordination modes, acting as a bidentate or tridentate ligand through its nitrogen atoms and/or the oxygen atom of the carbonyl group. Its ability to form chelate rings with metal ions enhances the stability of the resulting complexes. [, ]
Q4: What types of metal complexes does DPK form?
A4: DPK readily forms complexes with a wide array of transition metals, including copper, nickel, manganese, cobalt, palladium, and others. These complexes range from mononuclear to polynuclear structures, often exhibiting fascinating topologies. [, ]
Q5: What is the significance of the gem-diol and hemiacetal forms of DPK in coordination chemistry?
A5: The gem-diol form, (py)2C(OH)2, and the hemiacetal form, (py)2C(OR)(OH), generated in situ under certain conditions, can act as doubly and singly deprotonated ligands, respectively. These anionic forms exhibit versatile bridging modes, leading to the assembly of polynuclear metal clusters with intriguing structural motifs. [, , , ]
Q6: Can you give specific examples of metal complexes with DPK and their structural features?
A6: Certainly! [Cu4(dpk·CH3O)2Cl6]n represents a copper(II) polymer where a unimethylated diol derivative of DPK bridges copper ions, forming a tetranuclear repeating unit with an S = 2 ground state. [] Another notable example is a family of octanuclear Cu-Ln (Ln = lanthanide) complexes, [Cu6Ln2(tea)2{(py)2CO2}3Cl6]·xH2O, where the gem-diol form of DPK contributes to the formation of a unique structural motif. []
Q7: How does the structure of the DPK ligand influence the magnetic properties of its metal complexes?
A7: The nature of the bridging ligands derived from DPK, such as the gem-diolate or the oxime, significantly affects the magnetic exchange pathways between metal centers. Depending on the bridging mode and the geometry of the complex, DPK-based metal clusters can exhibit ferromagnetic or antiferromagnetic interactions. [, , , ]
Q8: Are there any applications of DPK-metal complexes in catalysis?
A8: Yes, certain palladium(II) complexes incorporating DPK have demonstrated promising catalytic activity in Suzuki cross-coupling reactions, enabling the formation of new carbon-carbon bonds. [] Additionally, copper(I) complexes of DPK have shown efficiency as photocatalysts in the conversion of norbornadiene to quadricyclane, a reaction relevant to solar energy storage. []
Q9: Have any computational studies been performed on DPK and its metal complexes?
A9: Computational studies, including density functional theory (DFT) calculations, have been employed to investigate the electronic structure, spectroscopic properties, and redox behavior of DPK-metal complexes. These calculations provide valuable insights into the nature of metal-ligand bonding and can aid in predicting the properties of new complexes. []
Q10: What analytical applications have been explored for DPK and its derivatives?
A10: DPK and its hydrazone derivatives have found applications as chromogenic and fluorogenic reagents for the spectrophotometric and spectrofluorimetric determination of various metal ions, including uranium, gallium, zinc, and palladium. [, , , , ]
Q11: What are the key advantages of using DPK-based reagents in analytical chemistry?
A11: DPK-based reagents often exhibit high sensitivity and selectivity for target metal ions. Their complexes typically display intense absorption or fluorescence bands, allowing for low detection limits. Additionally, the formation of these complexes is often rapid and occurs under mild conditions. []
Q12: Are there any known environmental concerns associated with this compound or its metal complexes?
A12: While specific studies on the environmental impact of DPK are limited, the potential ecotoxicological effects of DPK and its metal complexes, particularly those involving heavy metals, should be carefully considered. Appropriate waste management strategies and adherence to safety regulations are crucial when handling these compounds. []
Q13: What are some promising avenues for future research on this compound?
A13: Future research could focus on:
- Investigating the biological activity of DPK complexes: Given the promising antiproliferative activity observed for certain DPK-metal complexes, further exploration of their medicinal potential is warranted. []
- Developing sustainable synthetic methodologies: Designing environmentally benign routes for the synthesis of DPK and its derivatives aligns with the principles of green chemistry. []
Disclaimer and Information on In-Vitro Research Products
Please be aware that all articles and product information presented on BenchChem are intended solely for informational purposes. The products available for purchase on BenchChem are specifically designed for in-vitro studies, which are conducted outside of living organisms. In-vitro studies, derived from the Latin term "in glass," involve experiments performed in controlled laboratory settings using cells or tissues. It is important to note that these products are not categorized as medicines or drugs, and they have not received approval from the FDA for the prevention, treatment, or cure of any medical condition, ailment, or disease. We must emphasize that any form of bodily introduction of these products into humans or animals is strictly prohibited by law. It is essential to adhere to these guidelines to ensure compliance with legal and ethical standards in research and experimentation.