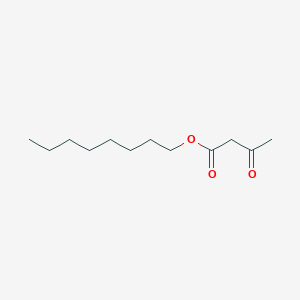
Acetoacetic Acid n-Octyl Ester
Overview
Description
Acetoacetic Acid n-Octyl Ester, also known as n-octyl acetoacetate, is an organic compound with the molecular formula C12H22O3. It is an ester formed from the reaction of octanol and acetoacetic acid. This compound is a colorless to light yellow liquid with a mild, pleasant odor. It is used in various industrial applications due to its unique chemical properties.
Mechanism of Action
Target of Action
Esters like octyl 3-oxobutanoate typically interact with enzymes that can hydrolyze the ester bond, such as esterases or lipases .
Mode of Action
Esters are known to undergo hydrolysis in the presence of water and enzymes, breaking down into their constituent alcohol and carboxylic acid . In the case of Octyl 3-oxobutanoate, it would break down into octanol and acetoacetic acid .
Biochemical Pathways
For instance, acetoacetic acid is a key intermediate in the metabolic pathway of ketone body metabolism .
Pharmacokinetics
As an ester, it is likely to be lipophilic, which could influence its absorption and distribution in the body .
Result of Action
For instance, acetoacetic acid can serve as an energy source for heart and brain cells .
Action Environment
Environmental factors such as pH, temperature, and the presence of specific enzymes can influence the action, efficacy, and stability of Octyl 3-oxobutanoate .
Preparation Methods
Synthetic Routes and Reaction Conditions: Acetoacetic Acid n-Octyl Ester is typically synthesized through the esterification of octanol with acetoacetic acid. The reaction is catalyzed by an acid, commonly sulfuric acid, under reflux conditions. The overall reaction can be represented as: [ \text{C}8\text{H}{17}\text{OH} + \text{CH}3\text{COCH}2\text{COOH} \rightarrow \text{C}{12}\text{H}{22}\text{O}_3 + \text{H}_2\text{O} ]
Industrial Production Methods: In industrial settings, the production of octyl acetoacetate involves the use of continuous reactors to maintain optimal reaction conditions and maximize yield. The process includes the removal of water formed during the reaction to drive the equilibrium towards the formation of the ester. The product is then purified through distillation.
Types of Reactions:
Esterification and Hydrolysis: this compound can undergo esterification and hydrolysis reactions. In the presence of water and an acid or base catalyst, it can be hydrolyzed back to octanol and acetoacetic acid.
Substitution Reactions: The compound can participate in nucleophilic substitution reactions, where the ester group can be replaced by other nucleophiles.
Reduction Reactions: this compound can be reduced to octyl alcohol and acetoacetate using reducing agents like lithium aluminum hydride.
Common Reagents and Conditions:
Acid Catalysts: Sulfuric acid, hydrochloric acid.
Base Catalysts: Sodium hydroxide, potassium hydroxide.
Reducing Agents: Lithium aluminum hydride, sodium borohydride.
Major Products Formed:
Hydrolysis: Octanol and acetoacetic acid.
Reduction: Octyl alcohol and acetoacetate.
Scientific Research Applications
Acetoacetic Acid n-Octyl Ester has a wide range of applications in scientific research:
Chemistry: It is used as a reagent in organic synthesis, particularly in the preparation of β-keto esters and other complex molecules.
Biology: The compound is used in the study of enzyme-catalyzed reactions and metabolic pathways involving esters.
Medicine: this compound is explored for its potential use in drug delivery systems due to its ability to form stable complexes with various drugs.
Industry: It is used as a solvent in the formulation of paints, coatings, and adhesives. Additionally, it serves as a plasticizer in the production of flexible plastics.
Comparison with Similar Compounds
- Ethyl acetoacetate
- Methyl acetoacetate
- Butyl acetoacetate
Properties
IUPAC Name |
octyl 3-oxobutanoate | |
---|---|---|
Source | PubChem | |
URL | https://pubchem.ncbi.nlm.nih.gov | |
Description | Data deposited in or computed by PubChem | |
InChI |
InChI=1S/C12H22O3/c1-3-4-5-6-7-8-9-15-12(14)10-11(2)13/h3-10H2,1-2H3 | |
Source | PubChem | |
URL | https://pubchem.ncbi.nlm.nih.gov | |
Description | Data deposited in or computed by PubChem | |
InChI Key |
IKYDDBGYKFPTGF-UHFFFAOYSA-N | |
Source | PubChem | |
URL | https://pubchem.ncbi.nlm.nih.gov | |
Description | Data deposited in or computed by PubChem | |
Canonical SMILES |
CCCCCCCCOC(=O)CC(=O)C | |
Source | PubChem | |
URL | https://pubchem.ncbi.nlm.nih.gov | |
Description | Data deposited in or computed by PubChem | |
Molecular Formula |
C12H22O3 | |
Source | PubChem | |
URL | https://pubchem.ncbi.nlm.nih.gov | |
Description | Data deposited in or computed by PubChem | |
DSSTOX Substance ID |
DTXSID10167754 | |
Record name | Octyl acetoacetate | |
Source | EPA DSSTox | |
URL | https://comptox.epa.gov/dashboard/DTXSID10167754 | |
Description | DSSTox provides a high quality public chemistry resource for supporting improved predictive toxicology. | |
Molecular Weight |
214.30 g/mol | |
Source | PubChem | |
URL | https://pubchem.ncbi.nlm.nih.gov | |
Description | Data deposited in or computed by PubChem | |
CAS No. |
16436-00-3 | |
Record name | Octyl acetoacetate | |
Source | CAS Common Chemistry | |
URL | https://commonchemistry.cas.org/detail?cas_rn=16436-00-3 | |
Description | CAS Common Chemistry is an open community resource for accessing chemical information. Nearly 500,000 chemical substances from CAS REGISTRY cover areas of community interest, including common and frequently regulated chemicals, and those relevant to high school and undergraduate chemistry classes. This chemical information, curated by our expert scientists, is provided in alignment with our mission as a division of the American Chemical Society. | |
Explanation | The data from CAS Common Chemistry is provided under a CC-BY-NC 4.0 license, unless otherwise stated. | |
Record name | Octyl acetoacetate | |
Source | ChemIDplus | |
URL | https://pubchem.ncbi.nlm.nih.gov/substance/?source=chemidplus&sourceid=0016436003 | |
Description | ChemIDplus is a free, web search system that provides access to the structure and nomenclature authority files used for the identification of chemical substances cited in National Library of Medicine (NLM) databases, including the TOXNET system. | |
Record name | Octyl acetoacetate | |
Source | EPA DSSTox | |
URL | https://comptox.epa.gov/dashboard/DTXSID10167754 | |
Description | DSSTox provides a high quality public chemistry resource for supporting improved predictive toxicology. | |
Record name | Octyl acetoacetate | |
Source | European Chemicals Agency (ECHA) | |
URL | https://echa.europa.eu/substance-information/-/substanceinfo/100.036.794 | |
Description | The European Chemicals Agency (ECHA) is an agency of the European Union which is the driving force among regulatory authorities in implementing the EU's groundbreaking chemicals legislation for the benefit of human health and the environment as well as for innovation and competitiveness. | |
Explanation | Use of the information, documents and data from the ECHA website is subject to the terms and conditions of this Legal Notice, and subject to other binding limitations provided for under applicable law, the information, documents and data made available on the ECHA website may be reproduced, distributed and/or used, totally or in part, for non-commercial purposes provided that ECHA is acknowledged as the source: "Source: European Chemicals Agency, http://echa.europa.eu/". Such acknowledgement must be included in each copy of the material. ECHA permits and encourages organisations and individuals to create links to the ECHA website under the following cumulative conditions: Links can only be made to webpages that provide a link to the Legal Notice page. | |
Record name | OCTYL ACETOACETATE | |
Source | FDA Global Substance Registration System (GSRS) | |
URL | https://gsrs.ncats.nih.gov/ginas/app/beta/substances/PL4K8GV3K4 | |
Description | The FDA Global Substance Registration System (GSRS) enables the efficient and accurate exchange of information on what substances are in regulated products. Instead of relying on names, which vary across regulatory domains, countries, and regions, the GSRS knowledge base makes it possible for substances to be defined by standardized, scientific descriptions. | |
Explanation | Unless otherwise noted, the contents of the FDA website (www.fda.gov), both text and graphics, are not copyrighted. They are in the public domain and may be republished, reprinted and otherwise used freely by anyone without the need to obtain permission from FDA. Credit to the U.S. Food and Drug Administration as the source is appreciated but not required. | |
Synthesis routes and methods
Procedure details
Retrosynthesis Analysis
AI-Powered Synthesis Planning: Our tool employs the Template_relevance Pistachio, Template_relevance Bkms_metabolic, Template_relevance Pistachio_ringbreaker, Template_relevance Reaxys, Template_relevance Reaxys_biocatalysis model, leveraging a vast database of chemical reactions to predict feasible synthetic routes.
One-Step Synthesis Focus: Specifically designed for one-step synthesis, it provides concise and direct routes for your target compounds, streamlining the synthesis process.
Accurate Predictions: Utilizing the extensive PISTACHIO, BKMS_METABOLIC, PISTACHIO_RINGBREAKER, REAXYS, REAXYS_BIOCATALYSIS database, our tool offers high-accuracy predictions, reflecting the latest in chemical research and data.
Strategy Settings
Precursor scoring | Relevance Heuristic |
---|---|
Min. plausibility | 0.01 |
Model | Template_relevance |
Template Set | Pistachio/Bkms_metabolic/Pistachio_ringbreaker/Reaxys/Reaxys_biocatalysis |
Top-N result to add to graph | 6 |
Feasible Synthetic Routes
Q1: What is the relevance of studying octyl acetoacetate under high-pressure CO2?
A1: The study explores the thermodynamic properties of octyl acetoacetate in the presence of high-pressure CO2 []. This is relevant because supercritical CO2 is increasingly employed as a green solvent in various industrial processes, including extraction, reaction, and material processing. Understanding the behavior of compounds like octyl acetoacetate in such environments is crucial for optimizing these processes and developing new applications.
Disclaimer and Information on In-Vitro Research Products
Please be aware that all articles and product information presented on BenchChem are intended solely for informational purposes. The products available for purchase on BenchChem are specifically designed for in-vitro studies, which are conducted outside of living organisms. In-vitro studies, derived from the Latin term "in glass," involve experiments performed in controlled laboratory settings using cells or tissues. It is important to note that these products are not categorized as medicines or drugs, and they have not received approval from the FDA for the prevention, treatment, or cure of any medical condition, ailment, or disease. We must emphasize that any form of bodily introduction of these products into humans or animals is strictly prohibited by law. It is essential to adhere to these guidelines to ensure compliance with legal and ethical standards in research and experimentation.