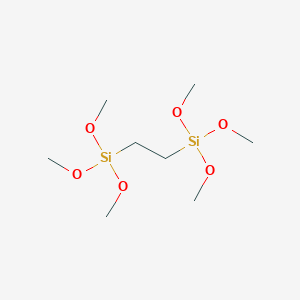
1,2-Bis(trimethoxysilyl)ethane
Overview
Description
1,2-Bis(trimethoxysilyl)ethane is an organosilicon compound with the molecular formula C8H22O6Si2. It is a bridged alkoxysilane that serves as a precursor for the synthesis of mesoporous materials. This compound is known for its ability to form Si-O-Si linkages, making it valuable in various applications, including the creation of hybrid materials and coatings .
Mechanism of Action
Target of Action
1,2-Bis(trimethoxysilyl)ethane (BTME) is a bridged alkoxysilane that primarily targets silica networks . It is used as an organosilica-based precursor in the preparation of mesoporous materials .
Mode of Action
BTME interacts with its targets by hydrolyzing the alkoxy groups with Si-OH to form Si-O-Si structures . This interaction results in the formation of mesoporous materials .
Biochemical Pathways
The primary biochemical pathway affected by BTME involves the formation of mesoporous materials. The hydrolysis of the alkoxy groups leads to the formation of Si-O-Si structures, which are key components of these materials .
Pharmacokinetics
It has high solubility and can dissolve in organic solvents such as ethanol, acetone, and dimethylformamide .
Result of Action
The molecular and cellular effects of BTME’s action primarily involve the formation of mesoporous materials. These materials have a wide range of applications, including molecular separation . BTME can also be used to synthesize a shell-based nanocomposite for potential application in drug delivery and encapsulation . It can also form magnetic hollow spheres which find usage in magnetic resonance imaging (MRI) .
Action Environment
The action, efficacy, and stability of BTME can be influenced by environmental factors. For instance, it reacts slowly with moisture or water . Therefore, it should be stored and handled in a dry environment to prevent unwanted reactions. Additionally, its boiling point is 103-104°C at 5mm Hg , indicating that it can be volatile at high temperatures. Therefore, temperature control is crucial when handling BTME.
Preparation Methods
1,2-Bis(trimethoxysilyl)ethane can be synthesized through the hydrolysis and condensation of trimethoxysilane derivatives. The typical synthetic route involves the reaction of silicon hydrides with trimethoxysilane compounds under controlled conditions. The reaction is usually carried out in the presence of a catalyst to facilitate the formation of the Si-O-Si network .
Industrial production methods often involve the use of large-scale reactors where the reaction conditions, such as temperature and pressure, are carefully monitored to ensure high yield and purity of the product. The resulting compound is then purified through distillation or other separation techniques to remove any impurities .
Chemical Reactions Analysis
1,2-Bis(trimethoxysilyl)ethane undergoes several types of chemical reactions, including:
Hydrolysis and Condensation: The compound readily hydrolyzes in the presence of water to form silanol groups, which can further condense to form Si-O-Si linkages.
Substitution Reactions: It can participate in substitution reactions where the methoxy groups are replaced by other functional groups, leading to the formation of various derivatives.
Oxidation and Reduction: While less common, the compound can undergo oxidation and reduction reactions under specific conditions, altering its chemical structure and properties.
Common reagents used in these reactions include water, acids, bases, and various organic solvents. The major products formed from these reactions are typically siloxane-based materials with enhanced chemical and thermal stability .
Scientific Research Applications
1,2-Bis(trimethoxysilyl)ethane has a wide range of applications in scientific research:
Comparison with Similar Compounds
1,2-Bis(trimethoxysilyl)ethane can be compared with other similar compounds, such as:
1,2-Bis(triethoxysilyl)ethane: This compound has ethoxy groups instead of methoxy groups, which can influence its reactivity and the properties of the resulting materials.
1,4-Bis(trimethoxysilyl)benzene: Featuring a benzene ring, this compound offers different structural and chemical properties, making it suitable for specific applications.
Bis(trimethylsilyl)acetylene: This compound contains trimethylsilyl groups and an acetylene linkage, providing unique reactivity and applications.
The uniqueness of this compound lies in its ability to form highly stable and versatile Si-O-Si networks, making it a valuable precursor for a wide range of applications in materials science and industry .
Biological Activity
1,2-Bis(trimethoxysilyl)ethane (BTME) is a silane compound that has garnered attention for its unique chemical properties and potential applications in various fields, including catalysis, materials science, and biomedical engineering. This article explores the biological activity of BTME, highlighting its synthesis, mechanisms of action, and relevant case studies.
BTME is a bifunctional silane that can undergo hydrolysis and condensation reactions to form siloxane networks. Its structure allows for the incorporation of organic functionalities into silica-based materials, enhancing their properties. The synthesis typically involves the hydrolytic polycondensation of BTME in the presence of a catalyst, leading to the formation of silicate networks that can be further modified for specific applications .
Biological Activity
The biological activity of BTME has been investigated primarily in the context of its role as a coupling agent and its effects on material properties. Key findings include:
- Cell Adhesion and Biocompatibility : Studies have shown that BTME can enhance cell adhesion when used as a surface modifier on biomaterials. This is crucial for applications in tissue engineering and regenerative medicine .
- Antimicrobial Properties : BTME-modified surfaces have demonstrated antimicrobial activity, potentially due to the release of silanol groups that can interact with microbial membranes .
- Drug Delivery Systems : The incorporation of BTME in polymeric matrices has been explored for controlled drug delivery systems, where it aids in the formation of stable nanocarriers with improved drug loading capacity .
1. Enhanced Cell Adhesion on Silica-Based Scaffolds
A study investigated the effects of BTME on silica scaffolds used for bone tissue engineering. The results indicated a significant increase in osteoblast adhesion and proliferation on BTME-treated surfaces compared to untreated controls. This enhancement is attributed to improved surface chemistry and wettability provided by the silane modification .
2. Antimicrobial Coatings
Research into antimicrobial coatings utilizing BTME showed that surfaces treated with this compound exhibited reduced bacterial colonization. The study utilized a variety of bacterial strains and demonstrated that BTME-modified coatings could inhibit growth effectively, suggesting potential applications in medical devices .
3. Drug Delivery Applications
In another case study, researchers developed a drug delivery system using BTME as a crosslinking agent within polymeric nanoparticles. The system showed sustained release profiles and enhanced stability under physiological conditions, indicating its potential for therapeutic applications .
Data Table: Summary of Biological Activities
The biological activities associated with BTME can be attributed to several mechanisms:
- Surface Modification : By altering the surface properties of materials, BTME enhances cell-material interactions, which is vital for biocompatibility.
- Release of Bioactive Species : The hydrolysis of BTME can lead to the formation of reactive silanol groups that may exert antimicrobial effects or promote cellular responses.
- Network Formation : The ability to form siloxane networks allows for the incorporation of various functional groups, tailoring materials for specific biological applications.
Properties
IUPAC Name |
trimethoxy(2-trimethoxysilylethyl)silane | |
---|---|---|
Source | PubChem | |
URL | https://pubchem.ncbi.nlm.nih.gov | |
Description | Data deposited in or computed by PubChem | |
InChI |
InChI=1S/C8H22O6Si2/c1-9-15(10-2,11-3)7-8-16(12-4,13-5)14-6/h7-8H2,1-6H3 | |
Source | PubChem | |
URL | https://pubchem.ncbi.nlm.nih.gov | |
Description | Data deposited in or computed by PubChem | |
InChI Key |
JCGDCINCKDQXDX-UHFFFAOYSA-N | |
Source | PubChem | |
URL | https://pubchem.ncbi.nlm.nih.gov | |
Description | Data deposited in or computed by PubChem | |
Canonical SMILES |
CO[Si](CC[Si](OC)(OC)OC)(OC)OC | |
Source | PubChem | |
URL | https://pubchem.ncbi.nlm.nih.gov | |
Description | Data deposited in or computed by PubChem | |
Molecular Formula |
C8H22O6Si2 | |
Source | PubChem | |
URL | https://pubchem.ncbi.nlm.nih.gov | |
Description | Data deposited in or computed by PubChem | |
Related CAS |
168259-18-5 | |
Record name | 2,7-Dioxa-3,6-disilaoctane, 3,3,6,6-tetramethoxy-, homopolymer | |
Source | CAS Common Chemistry | |
URL | https://commonchemistry.cas.org/detail?cas_rn=168259-18-5 | |
Description | CAS Common Chemistry is an open community resource for accessing chemical information. Nearly 500,000 chemical substances from CAS REGISTRY cover areas of community interest, including common and frequently regulated chemicals, and those relevant to high school and undergraduate chemistry classes. This chemical information, curated by our expert scientists, is provided in alignment with our mission as a division of the American Chemical Society. | |
Explanation | The data from CAS Common Chemistry is provided under a CC-BY-NC 4.0 license, unless otherwise stated. | |
DSSTOX Substance ID |
DTXSID6066369 | |
Record name | 2,7-Dioxa-3,6-disilaoctane, 3,3,6,6-tetramethoxy- | |
Source | EPA DSSTox | |
URL | https://comptox.epa.gov/dashboard/DTXSID6066369 | |
Description | DSSTox provides a high quality public chemistry resource for supporting improved predictive toxicology. | |
Molecular Weight |
270.43 g/mol | |
Source | PubChem | |
URL | https://pubchem.ncbi.nlm.nih.gov | |
Description | Data deposited in or computed by PubChem | |
Physical Description |
Colorless liquid; [Aldrich MSDS] | |
Record name | Hexamethoxydisilylethane | |
Source | Haz-Map, Information on Hazardous Chemicals and Occupational Diseases | |
URL | https://haz-map.com/Agents/9883 | |
Description | Haz-Map® is an occupational health database designed for health and safety professionals and for consumers seeking information about the adverse effects of workplace exposures to chemical and biological agents. | |
Explanation | Copyright (c) 2022 Haz-Map(R). All rights reserved. Unless otherwise indicated, all materials from Haz-Map are copyrighted by Haz-Map(R). No part of these materials, either text or image may be used for any purpose other than for personal use. Therefore, reproduction, modification, storage in a retrieval system or retransmission, in any form or by any means, electronic, mechanical or otherwise, for reasons other than personal use, is strictly prohibited without prior written permission. | |
Vapor Pressure |
0.08 [mmHg] | |
Record name | Hexamethoxydisilylethane | |
Source | Haz-Map, Information on Hazardous Chemicals and Occupational Diseases | |
URL | https://haz-map.com/Agents/9883 | |
Description | Haz-Map® is an occupational health database designed for health and safety professionals and for consumers seeking information about the adverse effects of workplace exposures to chemical and biological agents. | |
Explanation | Copyright (c) 2022 Haz-Map(R). All rights reserved. Unless otherwise indicated, all materials from Haz-Map are copyrighted by Haz-Map(R). No part of these materials, either text or image may be used for any purpose other than for personal use. Therefore, reproduction, modification, storage in a retrieval system or retransmission, in any form or by any means, electronic, mechanical or otherwise, for reasons other than personal use, is strictly prohibited without prior written permission. | |
CAS No. |
18406-41-2 | |
Record name | 1,2-Bis(trimethoxysilyl)ethane | |
Source | CAS Common Chemistry | |
URL | https://commonchemistry.cas.org/detail?cas_rn=18406-41-2 | |
Description | CAS Common Chemistry is an open community resource for accessing chemical information. Nearly 500,000 chemical substances from CAS REGISTRY cover areas of community interest, including common and frequently regulated chemicals, and those relevant to high school and undergraduate chemistry classes. This chemical information, curated by our expert scientists, is provided in alignment with our mission as a division of the American Chemical Society. | |
Explanation | The data from CAS Common Chemistry is provided under a CC-BY-NC 4.0 license, unless otherwise stated. | |
Record name | Hexamethoxydisilylethane | |
Source | ChemIDplus | |
URL | https://pubchem.ncbi.nlm.nih.gov/substance/?source=chemidplus&sourceid=0018406412 | |
Description | ChemIDplus is a free, web search system that provides access to the structure and nomenclature authority files used for the identification of chemical substances cited in National Library of Medicine (NLM) databases, including the TOXNET system. | |
Record name | 2,7-Dioxa-3,6-disilaoctane, 3,3,6,6-tetramethoxy- | |
Source | EPA Chemicals under the TSCA | |
URL | https://www.epa.gov/chemicals-under-tsca | |
Description | EPA Chemicals under the Toxic Substances Control Act (TSCA) collection contains information on chemicals and their regulations under TSCA, including non-confidential content from the TSCA Chemical Substance Inventory and Chemical Data Reporting. | |
Record name | 2,7-Dioxa-3,6-disilaoctane, 3,3,6,6-tetramethoxy- | |
Source | EPA DSSTox | |
URL | https://comptox.epa.gov/dashboard/DTXSID6066369 | |
Description | DSSTox provides a high quality public chemistry resource for supporting improved predictive toxicology. | |
Record name | 3,3,6,6-tetramethoxy-2,7-dioxa-3,6-disilaoctane | |
Source | European Chemicals Agency (ECHA) | |
URL | https://echa.europa.eu/substance-information/-/substanceinfo/100.038.426 | |
Description | The European Chemicals Agency (ECHA) is an agency of the European Union which is the driving force among regulatory authorities in implementing the EU's groundbreaking chemicals legislation for the benefit of human health and the environment as well as for innovation and competitiveness. | |
Explanation | Use of the information, documents and data from the ECHA website is subject to the terms and conditions of this Legal Notice, and subject to other binding limitations provided for under applicable law, the information, documents and data made available on the ECHA website may be reproduced, distributed and/or used, totally or in part, for non-commercial purposes provided that ECHA is acknowledged as the source: "Source: European Chemicals Agency, http://echa.europa.eu/". Such acknowledgement must be included in each copy of the material. ECHA permits and encourages organisations and individuals to create links to the ECHA website under the following cumulative conditions: Links can only be made to webpages that provide a link to the Legal Notice page. | |
Record name | 1,2-Bis(trimethoxysilyl)ethane | |
Source | FDA Global Substance Registration System (GSRS) | |
URL | https://gsrs.ncats.nih.gov/ginas/app/beta/substances/LJZ836PHD3 | |
Description | The FDA Global Substance Registration System (GSRS) enables the efficient and accurate exchange of information on what substances are in regulated products. Instead of relying on names, which vary across regulatory domains, countries, and regions, the GSRS knowledge base makes it possible for substances to be defined by standardized, scientific descriptions. | |
Explanation | Unless otherwise noted, the contents of the FDA website (www.fda.gov), both text and graphics, are not copyrighted. They are in the public domain and may be republished, reprinted and otherwise used freely by anyone without the need to obtain permission from FDA. Credit to the U.S. Food and Drug Administration as the source is appreciated but not required. | |
Retrosynthesis Analysis
AI-Powered Synthesis Planning: Our tool employs the Template_relevance Pistachio, Template_relevance Bkms_metabolic, Template_relevance Pistachio_ringbreaker, Template_relevance Reaxys, Template_relevance Reaxys_biocatalysis model, leveraging a vast database of chemical reactions to predict feasible synthetic routes.
One-Step Synthesis Focus: Specifically designed for one-step synthesis, it provides concise and direct routes for your target compounds, streamlining the synthesis process.
Accurate Predictions: Utilizing the extensive PISTACHIO, BKMS_METABOLIC, PISTACHIO_RINGBREAKER, REAXYS, REAXYS_BIOCATALYSIS database, our tool offers high-accuracy predictions, reflecting the latest in chemical research and data.
Strategy Settings
Precursor scoring | Relevance Heuristic |
---|---|
Min. plausibility | 0.01 |
Model | Template_relevance |
Template Set | Pistachio/Bkms_metabolic/Pistachio_ringbreaker/Reaxys/Reaxys_biocatalysis |
Top-N result to add to graph | 6 |
Feasible Synthetic Routes
Disclaimer and Information on In-Vitro Research Products
Please be aware that all articles and product information presented on BenchChem are intended solely for informational purposes. The products available for purchase on BenchChem are specifically designed for in-vitro studies, which are conducted outside of living organisms. In-vitro studies, derived from the Latin term "in glass," involve experiments performed in controlled laboratory settings using cells or tissues. It is important to note that these products are not categorized as medicines or drugs, and they have not received approval from the FDA for the prevention, treatment, or cure of any medical condition, ailment, or disease. We must emphasize that any form of bodily introduction of these products into humans or animals is strictly prohibited by law. It is essential to adhere to these guidelines to ensure compliance with legal and ethical standards in research and experimentation.