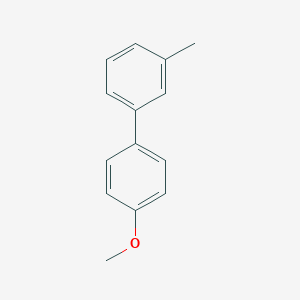
4-Methoxy-3'-methylbiphenyl
Overview
Description
Curdlan is an exopolysaccharide, specifically a homopolysaccharide, composed entirely of glucose molecules linked by β-1,3-glycosidic bonds . It is a high-molecular-weight polymer that forms elastic gels upon heating in aqueous suspension . Curdlan was first isolated in 1962 by Harada and his colleagues from the bacterium Alcaligenes faecalis var myxogenes . It has gained significant attention due to its unique physicochemical properties and its applications in various industries, including food, pharmaceuticals, and biotechnology .
Preparation Methods
Curdlan is primarily produced through microbial fermentation. The bacterium Alcaligenes faecalis var myxogenes is commonly used for its production . The production process involves submerged fermentation, where the bacteria are cultured in a nutrient-rich medium. Preliminary screening of curdlan-producing microorganisms is done using aniline blue media . To enhance the yield, various optimization techniques such as Plackett–Burman design and response surface methodology are employed .
Industrial production of curdlan involves the following steps:
Fermentation: The selected microorganism is cultured in a bioreactor under controlled conditions.
Harvesting: The biomass is harvested, and the curdlan is extracted.
Purification: The extracted curdlan is purified using techniques such as centrifugation and filtration.
Drying: The purified curdlan is dried to obtain a powder form.
Chemical Reactions Analysis
Curdlan undergoes various chemical reactions, including oxidation, reduction, and substitution. Some common reactions include:
Substitution: Curdlan can undergo substitution reactions to form derivatives such as carboxymethyl curdlan and sulfated curdlan.
The major products formed from these reactions include various curdlan derivatives with altered physicochemical properties, which can be used for specific applications .
Scientific Research Applications
Curdlan has a wide range of scientific research applications:
Mechanism of Action
Curdlan exerts its effects through various molecular mechanisms. One of the primary mechanisms involves the activation of the dectin-1 receptor on immune cells . This activation triggers a signaling cascade involving spleen tyrosine kinase (Syk), CARD9, and ERK pathways, leading to the modulation of immune responses . Curdlan’s ability to form gels and its unique physicochemical properties also contribute to its functionality in various applications .
Comparison with Similar Compounds
Curdlan is often compared with other polysaccharides such as xanthan, levan, and pullulan . While all these polysaccharides have unique properties, curdlan stands out due to its ability to form elastic gels upon heating and its high molecular weight . Additionally, curdlan’s β-1,3-glycosidic linkage is distinct from the linkages found in other polysaccharides, contributing to its unique properties .
Similar Compounds
Xanthan: A polysaccharide used as a thickening agent in food and industrial applications.
Levan: A fructan polysaccharide with applications in food and pharmaceuticals.
Pullulan: A polysaccharide used in food packaging and as a film-forming agent.
Curdlan’s unique properties and diverse applications make it a valuable compound in various fields of research and industry.
Properties
IUPAC Name |
1-methoxy-4-(3-methylphenyl)benzene | |
---|---|---|
Source | PubChem | |
URL | https://pubchem.ncbi.nlm.nih.gov | |
Description | Data deposited in or computed by PubChem | |
InChI |
InChI=1S/C14H14O/c1-11-4-3-5-13(10-11)12-6-8-14(15-2)9-7-12/h3-10H,1-2H3 | |
Source | PubChem | |
URL | https://pubchem.ncbi.nlm.nih.gov | |
Description | Data deposited in or computed by PubChem | |
InChI Key |
PGEFXSPLFJLFBA-UHFFFAOYSA-N | |
Source | PubChem | |
URL | https://pubchem.ncbi.nlm.nih.gov | |
Description | Data deposited in or computed by PubChem | |
Canonical SMILES |
CC1=CC(=CC=C1)C2=CC=C(C=C2)OC | |
Source | PubChem | |
URL | https://pubchem.ncbi.nlm.nih.gov | |
Description | Data deposited in or computed by PubChem | |
Molecular Formula |
C14H14O | |
Source | PubChem | |
URL | https://pubchem.ncbi.nlm.nih.gov | |
Description | Data deposited in or computed by PubChem | |
DSSTOX Substance ID |
DTXSID60374855 | |
Record name | 4-Methoxy-3'-methylbiphenyl | |
Source | EPA DSSTox | |
URL | https://comptox.epa.gov/dashboard/DTXSID60374855 | |
Description | DSSTox provides a high quality public chemistry resource for supporting improved predictive toxicology. | |
Molecular Weight |
198.26 g/mol | |
Source | PubChem | |
URL | https://pubchem.ncbi.nlm.nih.gov | |
Description | Data deposited in or computed by PubChem | |
CAS No. |
17171-17-4 | |
Record name | 4-Methoxy-3'-methylbiphenyl | |
Source | EPA DSSTox | |
URL | https://comptox.epa.gov/dashboard/DTXSID60374855 | |
Description | DSSTox provides a high quality public chemistry resource for supporting improved predictive toxicology. | |
Synthesis routes and methods
Procedure details
Retrosynthesis Analysis
AI-Powered Synthesis Planning: Our tool employs the Template_relevance Pistachio, Template_relevance Bkms_metabolic, Template_relevance Pistachio_ringbreaker, Template_relevance Reaxys, Template_relevance Reaxys_biocatalysis model, leveraging a vast database of chemical reactions to predict feasible synthetic routes.
One-Step Synthesis Focus: Specifically designed for one-step synthesis, it provides concise and direct routes for your target compounds, streamlining the synthesis process.
Accurate Predictions: Utilizing the extensive PISTACHIO, BKMS_METABOLIC, PISTACHIO_RINGBREAKER, REAXYS, REAXYS_BIOCATALYSIS database, our tool offers high-accuracy predictions, reflecting the latest in chemical research and data.
Strategy Settings
Precursor scoring | Relevance Heuristic |
---|---|
Min. plausibility | 0.01 |
Model | Template_relevance |
Template Set | Pistachio/Bkms_metabolic/Pistachio_ringbreaker/Reaxys/Reaxys_biocatalysis |
Top-N result to add to graph | 6 |
Feasible Synthetic Routes
Disclaimer and Information on In-Vitro Research Products
Please be aware that all articles and product information presented on BenchChem are intended solely for informational purposes. The products available for purchase on BenchChem are specifically designed for in-vitro studies, which are conducted outside of living organisms. In-vitro studies, derived from the Latin term "in glass," involve experiments performed in controlled laboratory settings using cells or tissues. It is important to note that these products are not categorized as medicines or drugs, and they have not received approval from the FDA for the prevention, treatment, or cure of any medical condition, ailment, or disease. We must emphasize that any form of bodily introduction of these products into humans or animals is strictly prohibited by law. It is essential to adhere to these guidelines to ensure compliance with legal and ethical standards in research and experimentation.