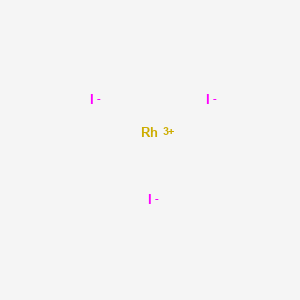
Rhodium triiodide
Overview
Description
Mechanism of Action
Target of Action
Rhodium(III) iodide, also known as Rhodium triiodide, is an inorganic compound with the formula RhI3 Some rhodium complexes have been found to interact with dna by inserting their moieties between the planar bases of b-dna through an intercalation mechanism .
Mode of Action
It is known that Rhodium(III) iodide can be synthesized by the reaction of aqueous potassium iodide with rhodium(III) bromide . The compound adopts the same crystal structure motif as AlCl3 and YCl3, consisting of cubic close-packed iodide ions and rhodium ions filling a third of the octahedral interstices .
Action Environment
Rhodium(III) iodide is only known in the anhydrous form . . This suggests that the compound’s action, efficacy, and stability may be influenced by environmental factors such as humidity.
Preparation Methods
Synthetic Routes and Reaction Conditions
The preparation of 3-aminosalicylic acid typically involves the nitration of salicylic acid to produce 3-nitrosalicylic acid, followed by catalytic hydrogenation reduction and dehalogenation hydrogenolysis reactions . The process can be summarized as follows:
Nitration: Salicylic acid is nitrated using a nitration agent to produce 3-nitrosalicylic acid.
Catalytic Hydrogenation Reduction: The 3-nitrosalicylic acid undergoes catalytic hydrogenation reduction in the presence of a catalyst and hydrogen donors.
Dehalogenation Hydrogenolysis: The final step involves dehalogenation hydrogenolysis to yield 3-aminosalicylic acid.
Industrial Production Methods
The industrial production of 3-aminosalicylic acid follows similar synthetic routes but is optimized for higher yields and cost-effectiveness. The use of cheaper raw materials and efficient catalysts is crucial for large-scale production .
Chemical Reactions Analysis
Types of Reactions
3-Aminosalicylic acid undergoes various chemical reactions, including:
Oxidation: It can be oxidized to form corresponding quinones.
Reduction: The nitro group in 3-nitrosalicylic acid can be reduced to an amine group.
Substitution: The amine and hydroxyl groups can participate in substitution reactions.
Common Reagents and Conditions
Oxidation: Common oxidizing agents include potassium permanganate (KMnO₄) and hydrogen peroxide (H₂O₂).
Reduction: Catalytic hydrogenation using palladium on carbon (Pd/C) as a catalyst.
Substitution: Reagents such as acyl chlorides and alkyl halides are used for substitution reactions.
Major Products Formed
Oxidation: Quinones and other oxidized derivatives.
Reduction: 3-Aminosalicylic acid from 3-nitrosalicylic acid.
Substitution: Various substituted derivatives depending on the reagents used.
Scientific Research Applications
3-Aminosalicylic acid has a wide range of applications in scientific research:
Comparison with Similar Compounds
Similar Compounds
2-Aminosalicylic acid: Another isomer with similar chemical properties but different biological activities.
Uniqueness
3-Aminosalicylic acid is unique due to its specific position of the amine group on the benzene ring, which imparts distinct chemical and biological properties. Its ability to inhibit folic acid synthesis makes it particularly effective as an anti-mycobacterial agent .
Properties
IUPAC Name |
rhodium(3+);triiodide | |
---|---|---|
Details | Computed by LexiChem 2.6.6 (PubChem release 2019.06.18) | |
Source | PubChem | |
URL | https://pubchem.ncbi.nlm.nih.gov | |
Description | Data deposited in or computed by PubChem | |
InChI |
InChI=1S/3HI.Rh/h3*1H;/q;;;+3/p-3 | |
Details | Computed by InChI 1.0.5 (PubChem release 2019.06.18) | |
Source | PubChem | |
URL | https://pubchem.ncbi.nlm.nih.gov | |
Description | Data deposited in or computed by PubChem | |
InChI Key |
KXAHUXSHRWNTOD-UHFFFAOYSA-K | |
Details | Computed by InChI 1.0.5 (PubChem release 2019.06.18) | |
Source | PubChem | |
URL | https://pubchem.ncbi.nlm.nih.gov | |
Description | Data deposited in or computed by PubChem | |
Canonical SMILES |
[Rh+3].[I-].[I-].[I-] | |
Details | Computed by OEChem 2.1.5 (PubChem release 2019.06.18) | |
Source | PubChem | |
URL | https://pubchem.ncbi.nlm.nih.gov | |
Description | Data deposited in or computed by PubChem | |
Molecular Formula |
I3Rh | |
Details | Computed by PubChem 2.1 (PubChem release 2019.06.18) | |
Source | PubChem | |
URL | https://pubchem.ncbi.nlm.nih.gov | |
Description | Data deposited in or computed by PubChem | |
Molecular Weight |
483.6189 g/mol | |
Details | Computed by PubChem 2.1 (PubChem release 2021.05.07) | |
Source | PubChem | |
URL | https://pubchem.ncbi.nlm.nih.gov | |
Description | Data deposited in or computed by PubChem | |
CAS No. |
15492-38-3 | |
Record name | Rhodium iodide (RhI3) | |
Source | CAS Common Chemistry | |
URL | https://commonchemistry.cas.org/detail?cas_rn=15492-38-3 | |
Description | CAS Common Chemistry is an open community resource for accessing chemical information. Nearly 500,000 chemical substances from CAS REGISTRY cover areas of community interest, including common and frequently regulated chemicals, and those relevant to high school and undergraduate chemistry classes. This chemical information, curated by our expert scientists, is provided in alignment with our mission as a division of the American Chemical Society. | |
Explanation | The data from CAS Common Chemistry is provided under a CC-BY-NC 4.0 license, unless otherwise stated. | |
Record name | Rhodium iodide (RhI3) | |
Source | ChemIDplus | |
URL | https://pubchem.ncbi.nlm.nih.gov/substance/?source=chemidplus&sourceid=0015492383 | |
Description | ChemIDplus is a free, web search system that provides access to the structure and nomenclature authority files used for the identification of chemical substances cited in National Library of Medicine (NLM) databases, including the TOXNET system. | |
Q1: What are the common methods for synthesizing Rhodium triiodide?
A: Several methods have been explored for synthesizing this compound. One approach involves reacting trivalent rhodium hydroxide with hydriodic acid at elevated temperatures (60-100°C). This method yields a cinereous and porous form of this compound that is easily filtered and boasts high purity with a rhodium content of about 19.5-21.5% []. Another method utilizes recovered rhodium from industrial waste streams, highlighting the potential for sustainable sourcing of this valuable compound [].
Q2: How does the structure of this compound contribute to its catalytic activity?
A2: While the provided research does not delve into the precise structural features contributing to its catalytic properties, it's known that RhI₃ can readily participate in various organometallic reactions. It can act as a Lewis acid, activating substrates through coordination. Additionally, the presence of iodide ligands can facilitate oxidative addition and reductive elimination steps, crucial for many catalytic cycles. Further research focusing on its crystal structure and electronic properties would be beneficial to gain a deeper understanding of its catalytic behavior.
Q3: Can you provide an example of a specific reaction catalyzed by this compound?
A: this compound has demonstrated promising activity in catalyzing carboamination reactions of alkynes []. This reaction allows for the formation of multi-substituted indoles, important heterocyclic compounds with various applications in medicinal and materials chemistry. Notably, the reaction proceeds with a low catalyst loading (5 mol% Rh), indicating high catalytic efficiency.
Q4: Are there any studies on the stability and material compatibility of this compound?
A: While the provided research does not explicitly discuss the stability and compatibility of this compound in detail, one study [] mentions a process involving the electrolysis of a solution containing nano-rhodium powder and potassium iodide in hydroiodic acid to produce this compound. This suggests that RhI₃ is stable under these specific electrolytic conditions. Further investigation into its stability in various solvents, temperatures, and in the presence of different reagents would be essential for its wider application in organic synthesis.
Q5: What are the potential applications of this compound in organic synthesis?
A: Based on current research, this compound holds significant promise as a catalyst for various organic transformations. Its ability to catalyze carboamination reactions [] showcases its potential in constructing complex nitrogen-containing molecules. Further exploration of its reactivity could reveal additional applications in C-C bond formation, C-H activation, and other valuable transformations.
Disclaimer and Information on In-Vitro Research Products
Please be aware that all articles and product information presented on BenchChem are intended solely for informational purposes. The products available for purchase on BenchChem are specifically designed for in-vitro studies, which are conducted outside of living organisms. In-vitro studies, derived from the Latin term "in glass," involve experiments performed in controlled laboratory settings using cells or tissues. It is important to note that these products are not categorized as medicines or drugs, and they have not received approval from the FDA for the prevention, treatment, or cure of any medical condition, ailment, or disease. We must emphasize that any form of bodily introduction of these products into humans or animals is strictly prohibited by law. It is essential to adhere to these guidelines to ensure compliance with legal and ethical standards in research and experimentation.